Bioremediation in the Wake of the Camp Fire
In the wake of the Camp Fire, California Office of Emergency Services was tasked with the clean-up of toxic ash and debris. Many properties were deemed ineligible for these services and left unaddressed. This document provides a few examples of some of these sites that were left undress, provides an overview of pollutants that were observed there and the effectiveness of fungi in remediation of those toxins.
Burned RV
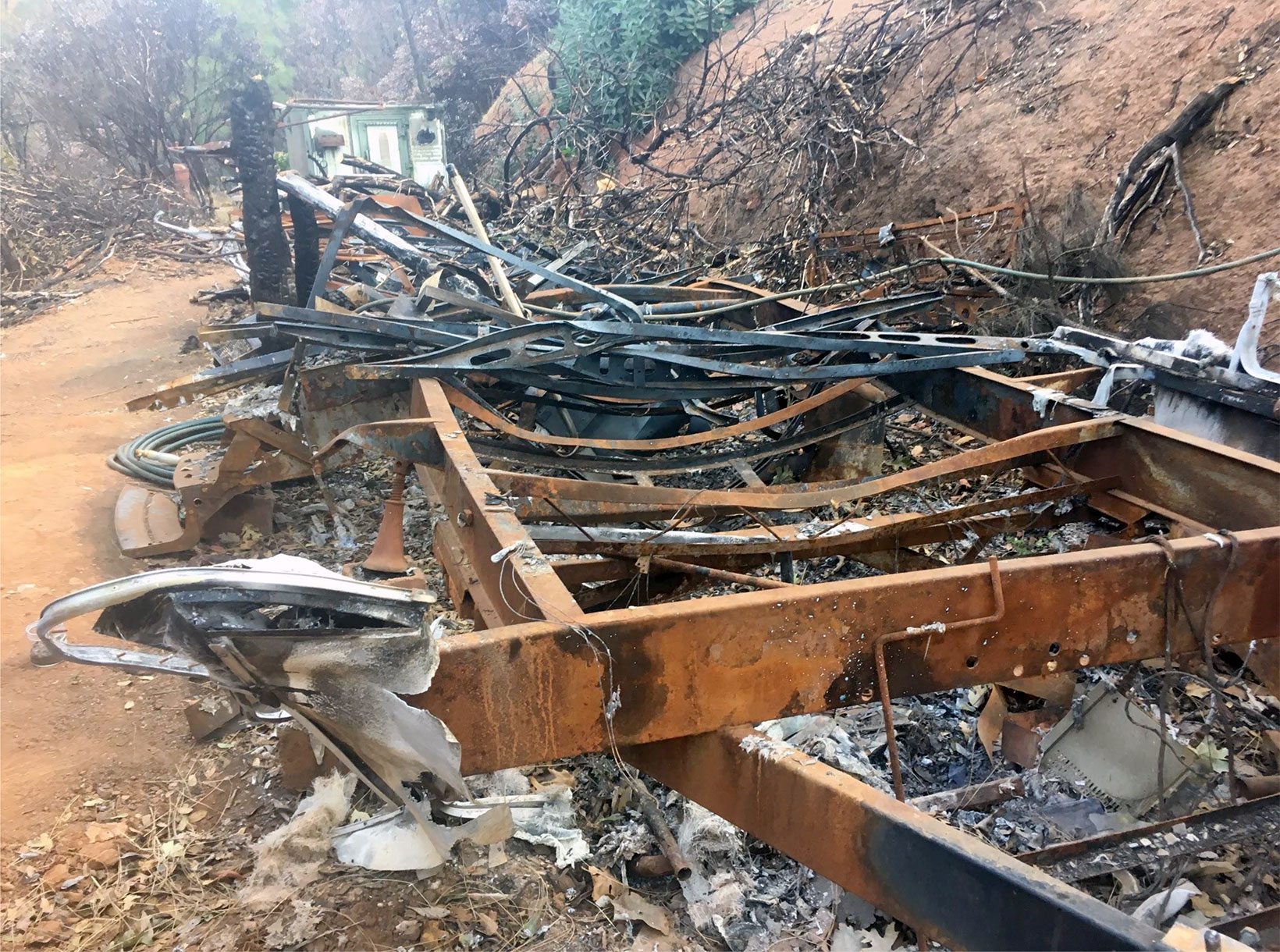
Figure 1. Burned Recreational Vehicle (RV). This RV served as the resident’s home prior to fire event and was determined ineligible for the Cal OES clean up program. Burn site was directly uphill from livestock and a creek presenting a pollutant risk to the environment.
In this situation the land owner was not eligible for the CalOES Cleanup (California Office of Emergency Services, Funded by FEMA) due to the fact that no actual structures had burned. The landowner was living in an RV, with no other major structures on the property. This RV was not considered a building over 120 sq. ft. and despite several vehicles and shipping containers had burned, no government clean-up support was provided.
The damage to the property was extensive. The property was seated on a steep hill with dense vegetation that had burned at very high temperatures. The RV itself was completely incinerated, leaving just the scorched frame. In addition, the frame was stuck behind two burnt shipping containers with no other access points for debris removal. Over a year after the fire event all the ashen debris remained in place.
A composite sampling of 5 different sites in the ash footprint of the RV were collected and tested for heavy metals, benzenes, dioxins, and PAHs. Heavy metals and dioxins were detected.
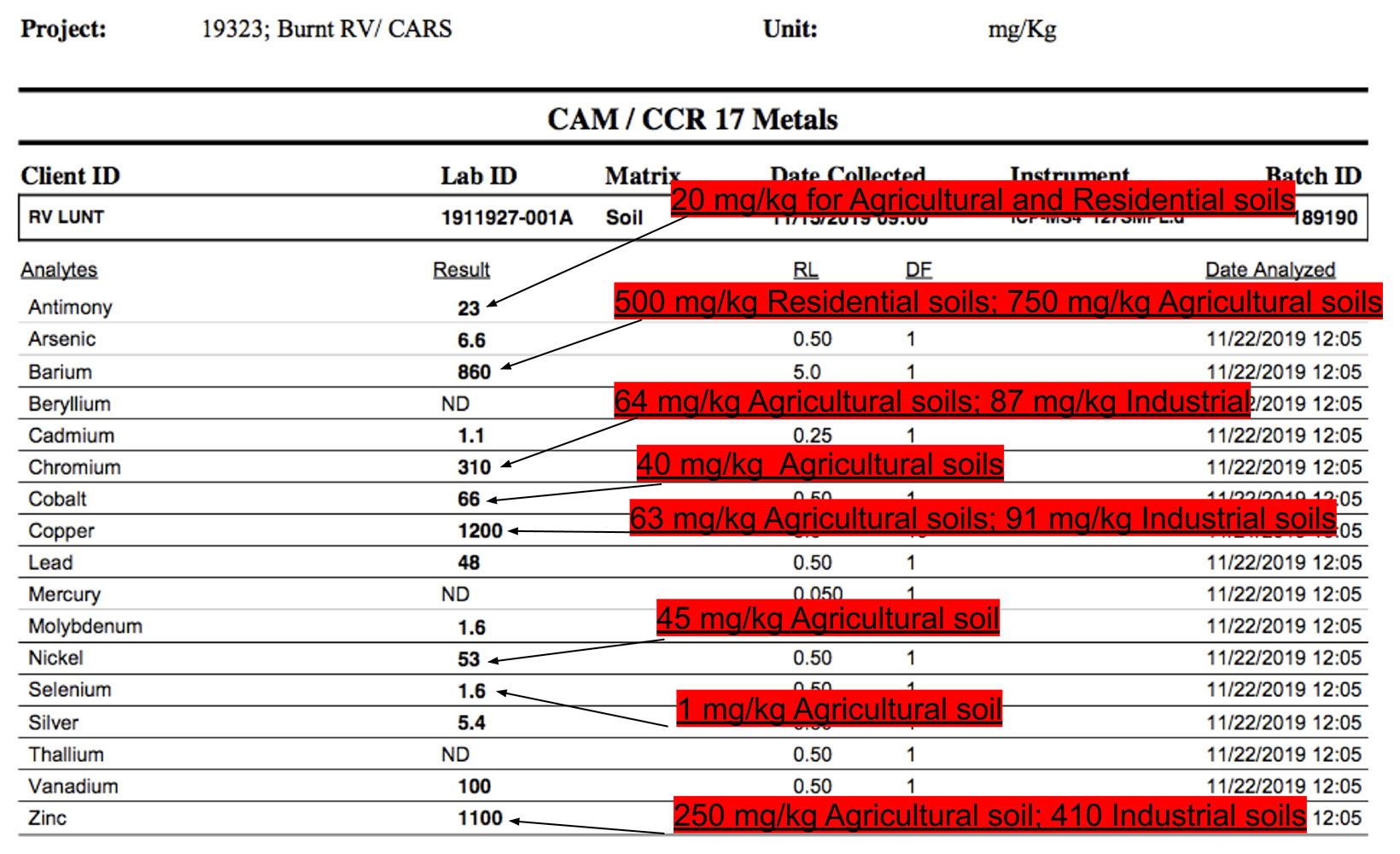
Figure 2. Heavy metal values for the burned RV reported on the basis of mg/kg on dry weight basis. Red highlights have the threshold for safe and allowable levels based on Canadian Council of Ministers of the Environment standards. Most notable values above standard are chromium, copper, barium, and zinc.
For the purpose of this project Oyster Mushroom (Pleurotus ostreatus) mushroom “spawn bags” were used. “Spawn bag” is the mushroom farming industry term for straw/woodchip filled bags with the fungus growing in it. This mushroom spawn was incorporated into debris by hand and top dressed with a layer of Jute Landscape Fabric and straw that had been sterilized in calcium hydroxide. These layers served for moisture protection, but also as an indicator of active mycelial growth.
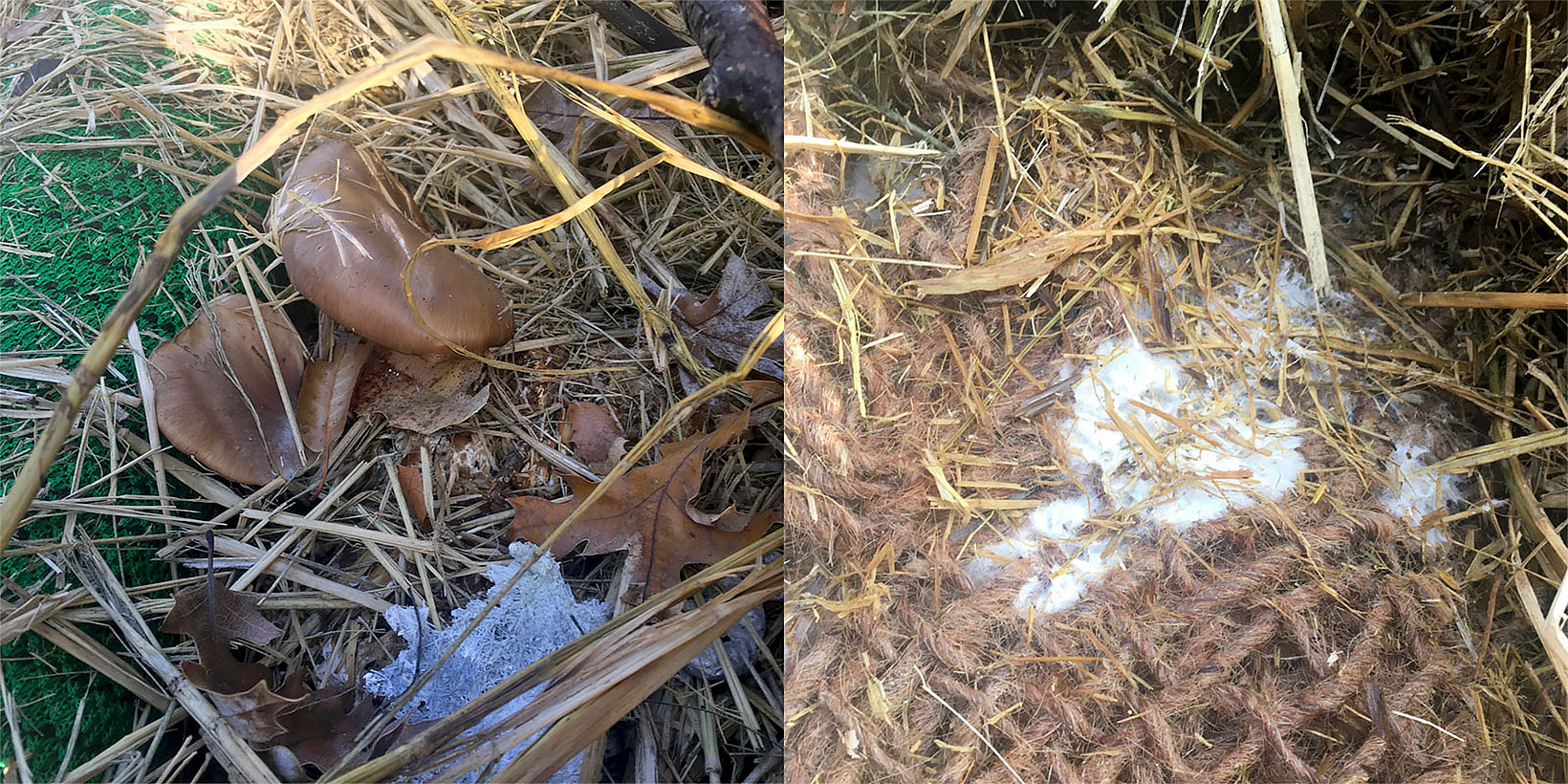
Figure 3. Mycelial and mushroom growth in ashen debris of RV frame (left) and fungi growing through Jute Landscape Fabric.
Fungus was permitted to grow for a duration of ~6 months, during which it was monitored for active mycelial growth and mushroom emergence. After this period the soil was resampled for contaminates for comparison to baseline levels.
This ash footprint had tested positive for dioxins. The cost of testing for dioxins is high, so laboratories typically only test for 2,3,7,8 -Tetrachlorodibenzo-p-dioxin (TCDD). TCDD values are reported in Technical Equivalent Factors (TEF’s or TEQ’s). The reason for this is that the presence of TCDD implies the presence of several other species/types of dioxins.
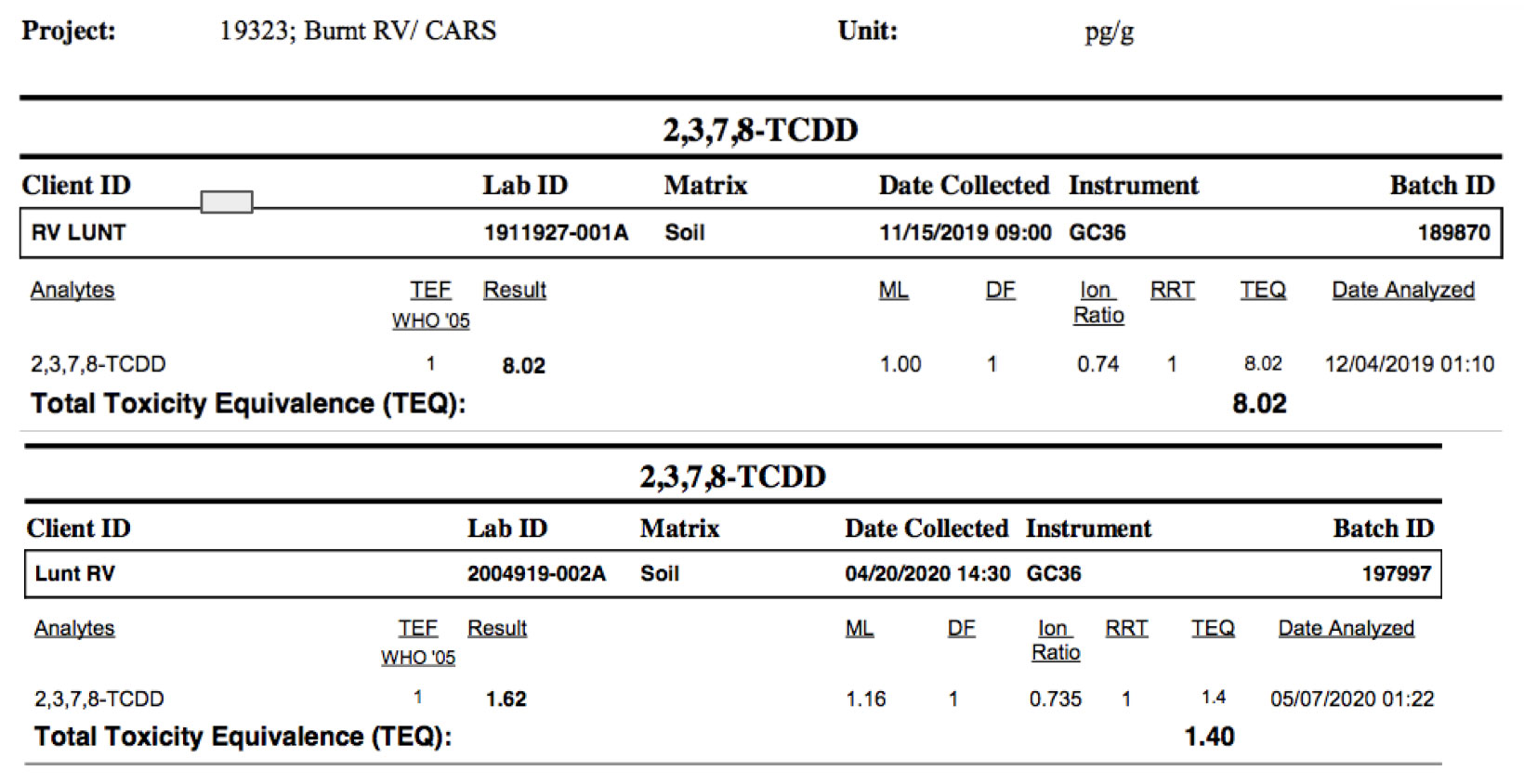
Figure 4. TEQ Values for debris before (above) and after (below) mycelial treatment. As above, initial TEQ is reported as 8.02, and after treatment TEQ values have been reduced to 1.62.
In 2005 the World Health organization set the safe and allowable threshold for TEFs/TEQs at 1.0. As seen here the initial toxic ash had a value 8 times that. Over the duration of the treatment there was a nearly 8 fold reduction in TEQ value. While it is possible that the dioxins could have precipitated further into the soil over the duration of the treatment, this RV was placed in a site with particularly poor drainage, and the natural half-life of dioxin is considered to be 8 years. This circumstance offers an encouraging example of the potential for fungi to reduce the toxicity of ashen debris prior to removal and disposal.
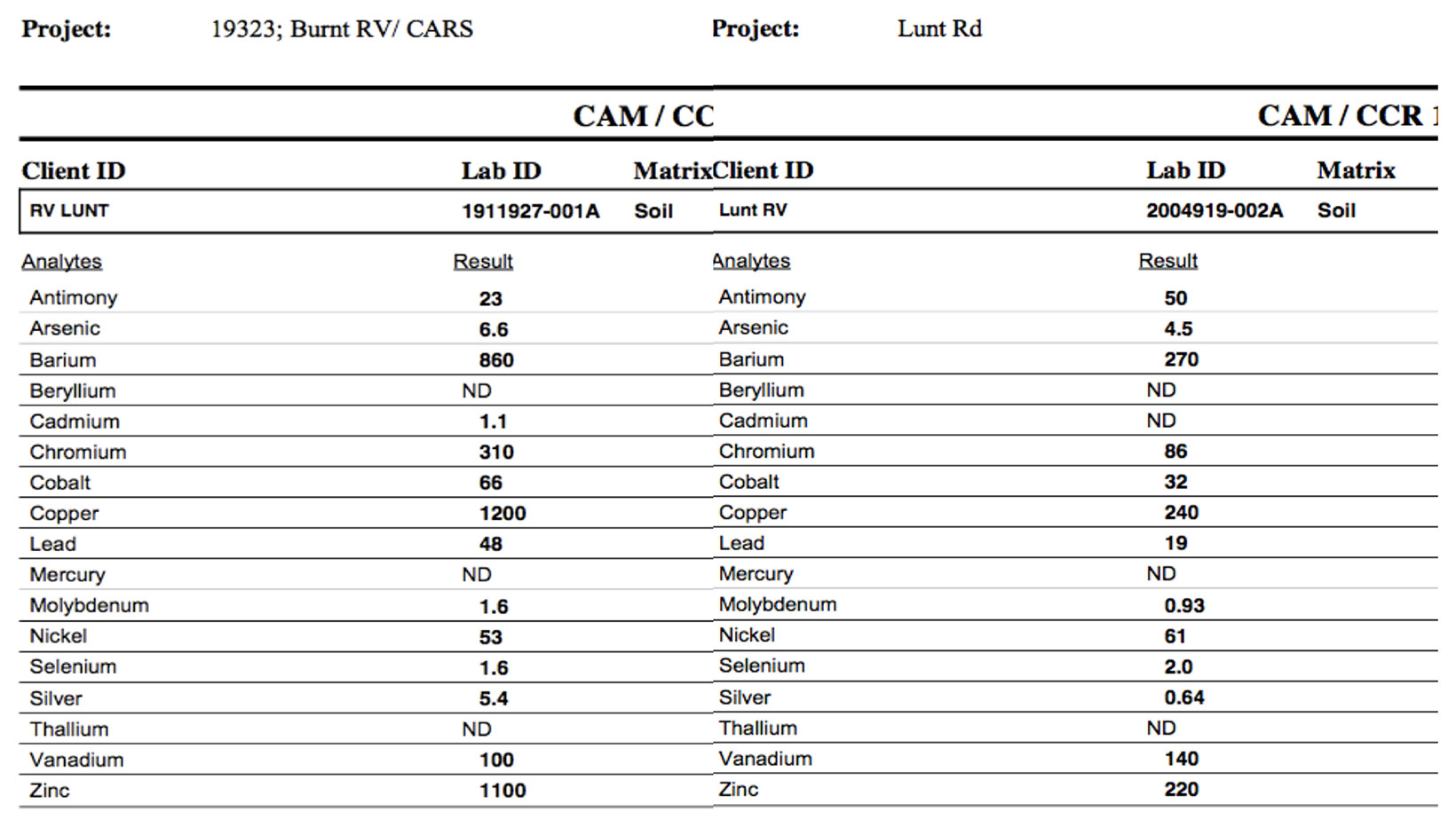
Figure 5. Heavy metal rates before (left) and after (right) treatment reported in mg/kg dry weight. Most notable values above standard (chromium, copper, barium, and zinc) have had significant reductions.
Over the span of the treatment heavy metal values were reduced for most ions. Most noteworthy are barium (near 3X reduction), chromium (over 3X reduction), copper (5X reduction), and zinc (5X reduction). It is worth pointing out the doubling of antimony values from 23 mg/kg to 50 mg/kg between samples. There are several possible explanations for this including sampling error (one particular samples site had a granule of antimony heavy soil), or possible mineralization of toxic metal due to the continued breakdown of toxic materials.
Burned Cars
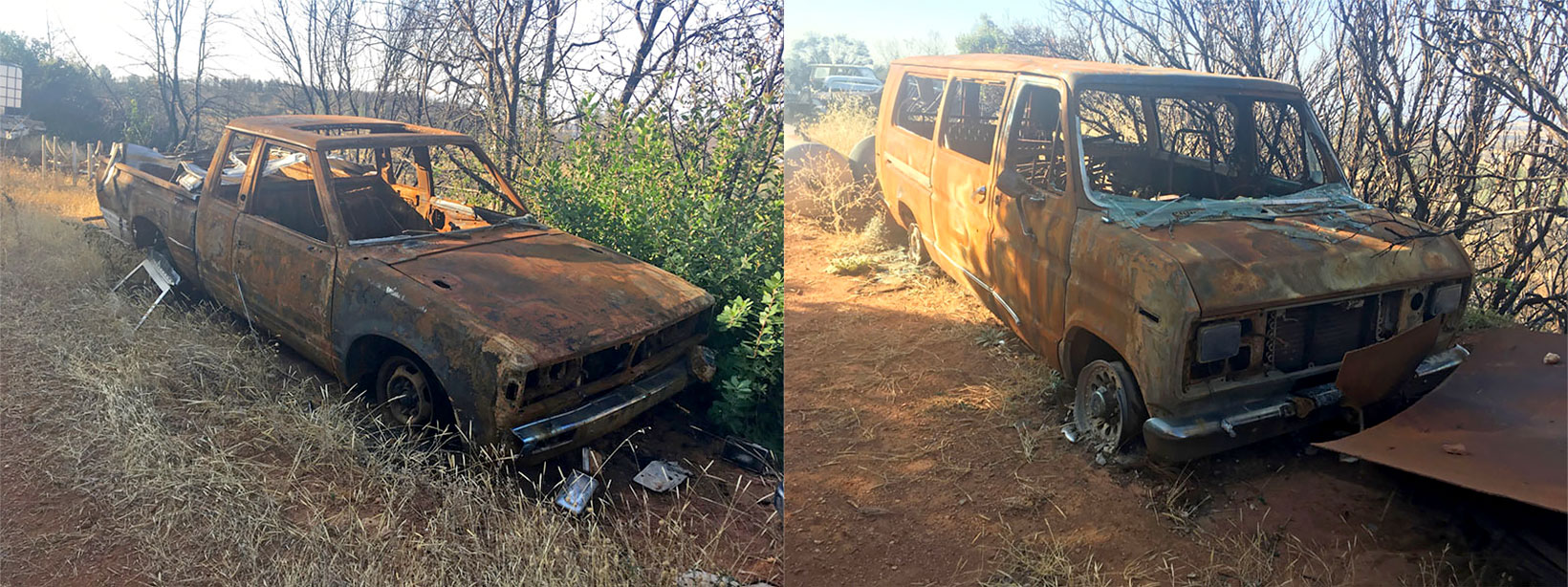
On the same site as the RV described above, there were cars were on a property that were ineligible for CalOES debris removal. In this circumstance there were several burned vehicles, in a remote section of forest, up a steep, rutted dirt road. As it is unlikely that a wrecking truck would be able to traverse or turn around on this narrow road, these vehicles would have to be dismantled and removed as scrap.
A composite sampling of 5 different sites on the downhill side of these vehicles were collected, and tested for heavy metals, benzenes, dioxins, and PAHs. Only heavy metals were detected.
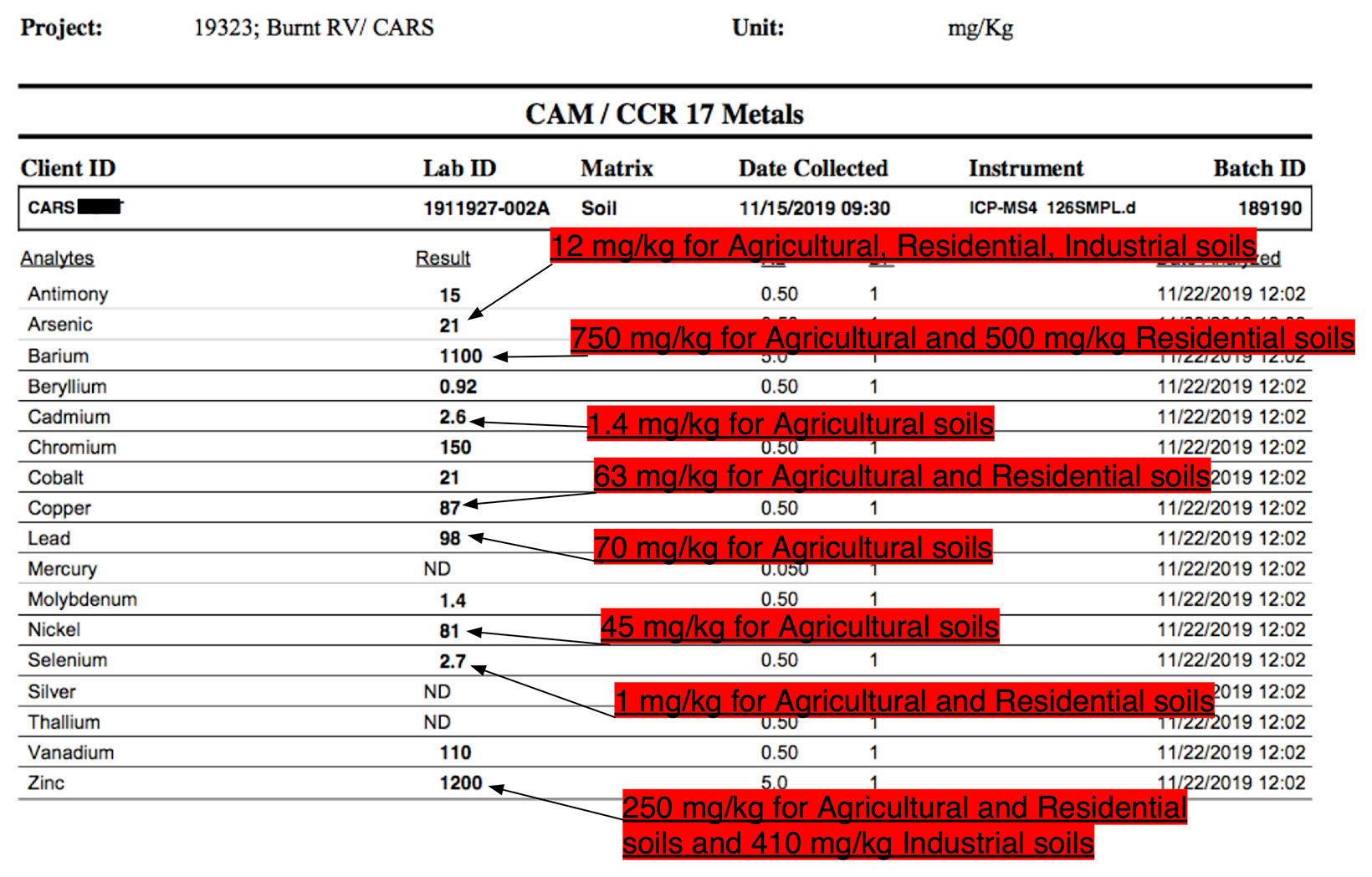
Figure 6. Heavy metal composite sample from several of the burned vehicles reported in mg/kg dry weight. Red highlights have the threshold for safe and allowable levels based on Canadian Council of Ministers of the Environment standards. Areas of concern include arsenic, barium, cadmium, chromium, nickel, lead, and zinc.
Similar to the methods described in the circumstance of the RV, mushroom spawn was incorporated into the ash footprint of the vehicles and top dressed with jute. Wattles (straw tubes used for erosion control) were placed on the downhill from the vehicles to try to minimize surface flow of contamination.
Fungus was permitted to grow for a duration of ~6 months, during which it was monitored for active mycelial growth and mushroom emergence. After this period the soil was resampled for contaminates for comparison to baseline levels.
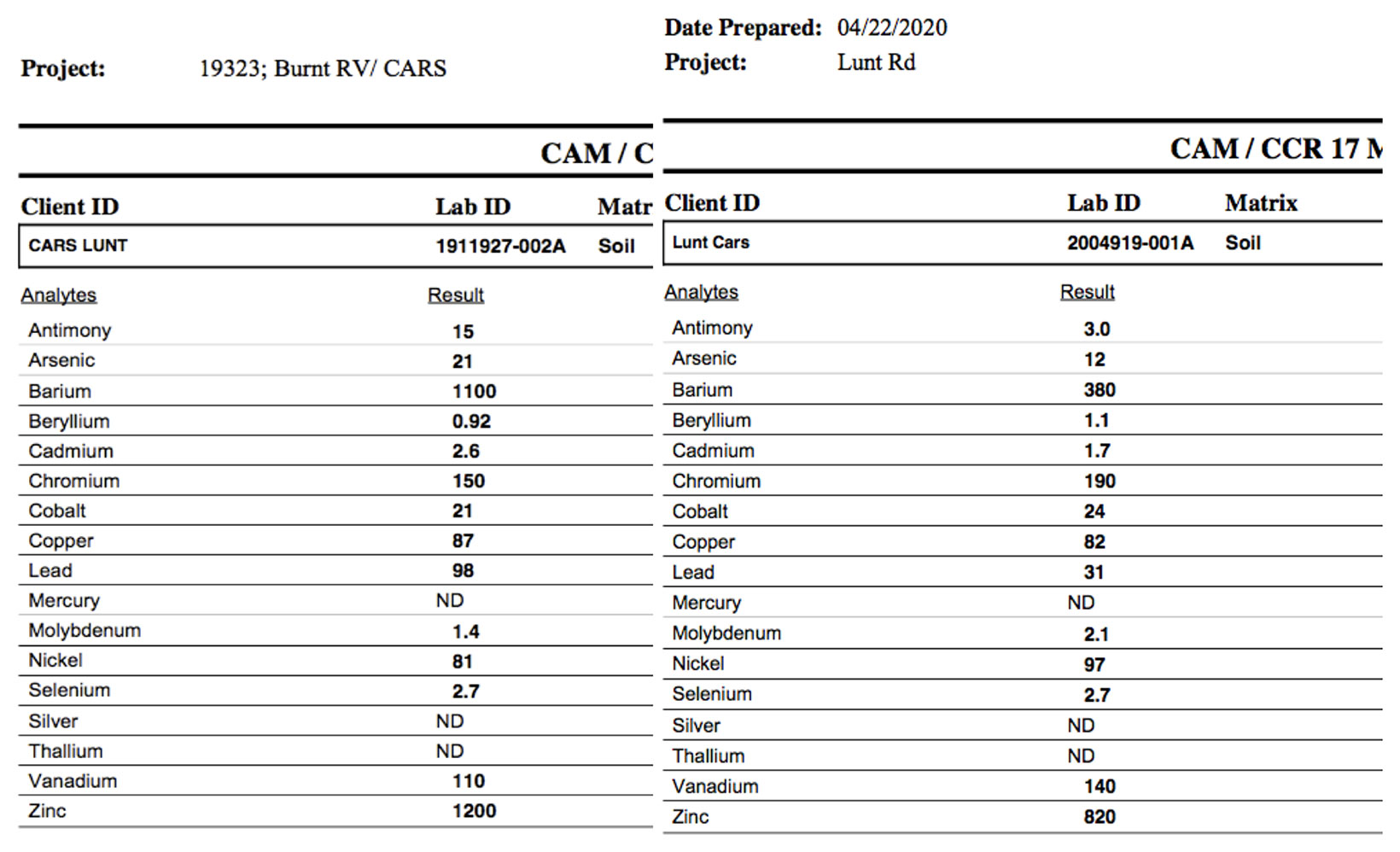
Figure 7. Before (left) and after (right) analysis of burned vehicle composite samples. While there are some promising results with reduction of antimony (5X reduction), barium (2X reduction), and perhaps most importantly lead (3X reduction), there is also several slight increases in toxin content of soils for several elements. Most noteworthy is chromium, which may be the result of further flaking off of chrome trim from the vehicles.
Burned RV
In this situation, another recreational vehicle had been incinerated. The frame of the RV had been removed but the debris of the chassis remained. The RV had been parked on a hard gravel pad and incorporation of fungal materials into the debris was difficult.
Ash footprint was sampled in several locations and soil tests revealed the presence of heavy metals and polycyclic aromatic hydrocarbons. Again, Oyster mushroom spawn was sheet mulched across the area and covered with jute landscape fabric and straw sterilized with calcium hydroxide.
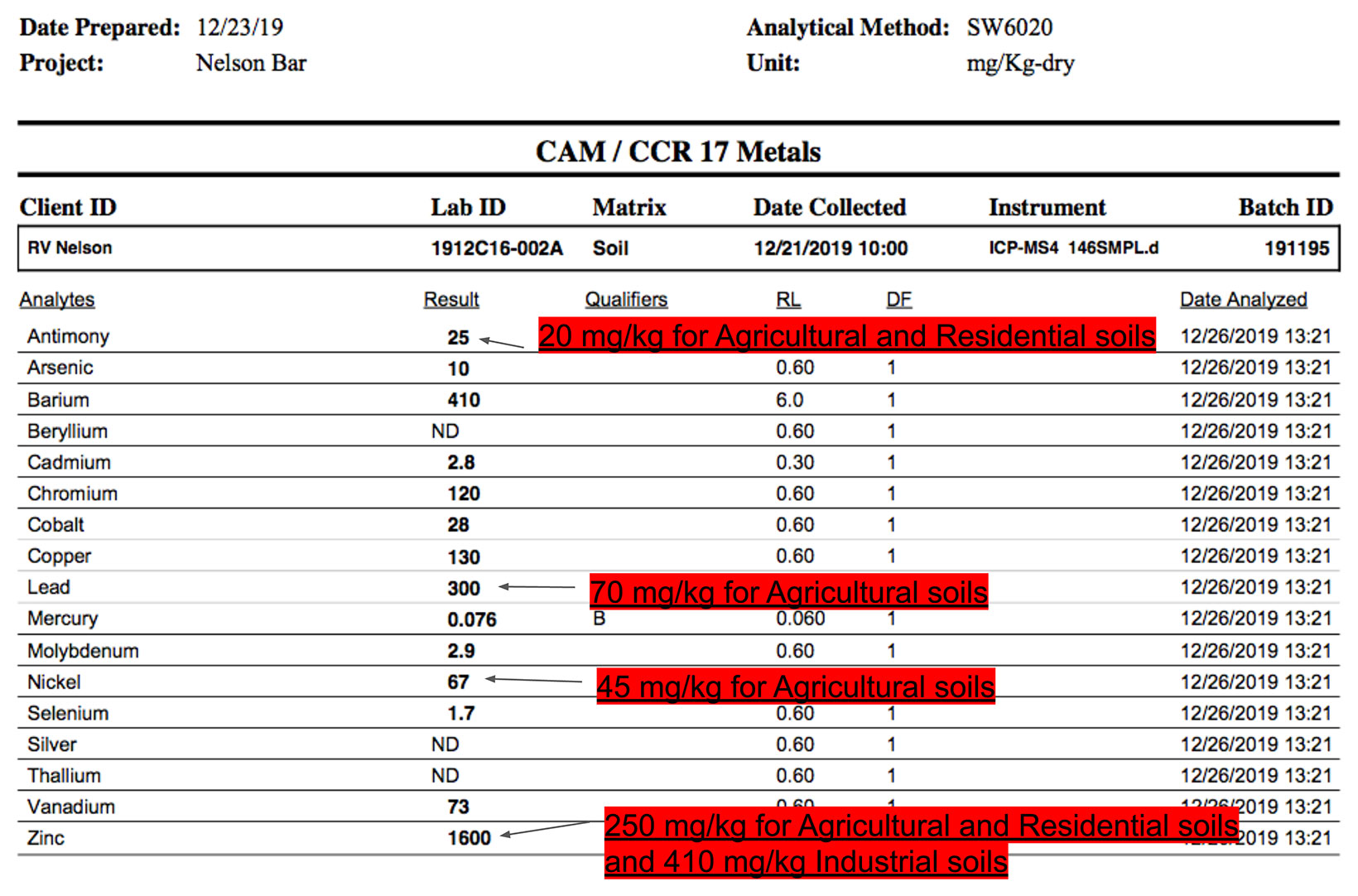
Figure 8. Heavy metals reported in mg/kg on a dry weight basis. Initial samples showed spikes in some heavy metals, specifically antimony, lead, nickel and zinc.
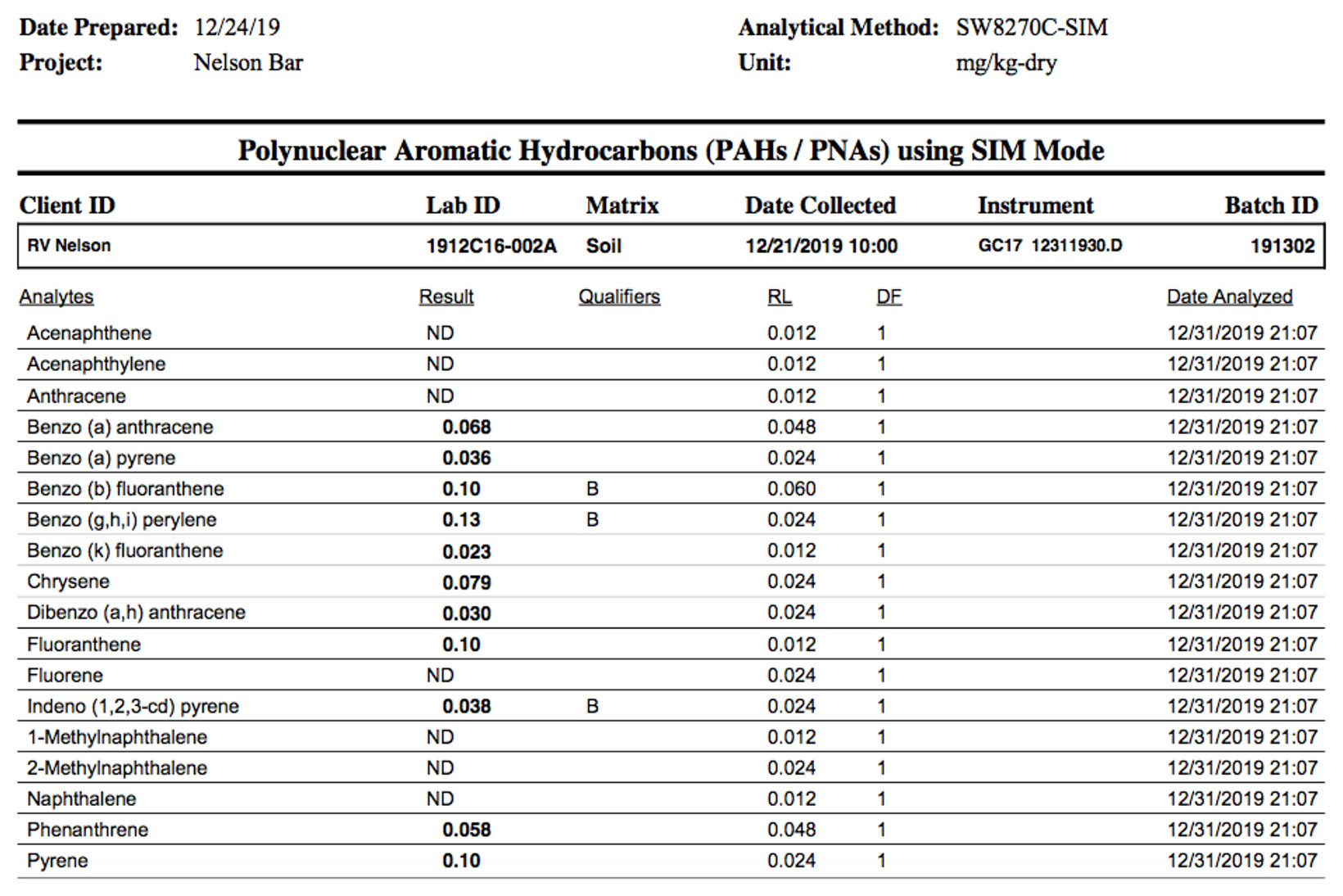
Figure 9. Polycyclic Aromatic Hydrocarbons reported in mg/kg. Both EPA and the Canadian CME standards for PAH toxicity are variable. Depending on exposure rate and land use safety standards change, and no solid number for what is safe and allowable was available.That said, the cumulative rates of PAH contamination seen here would be of some concern.
After a treatment period of 6 months soils were resampled. While results showed the polycyclic aromatic hydrocarbons had been completely ameliorated. It should be noted that initial samples were taken over a year after the fire event (speaking to the persistence of PAHs), and with a 6 month fungal treatment the persistent pollutants had been eliminated.
Conversely some heavy metals levels were actually increased over the duration of the of the treatment. This is possible due to either further degradation of ashen debris or hot spots in sample sites.
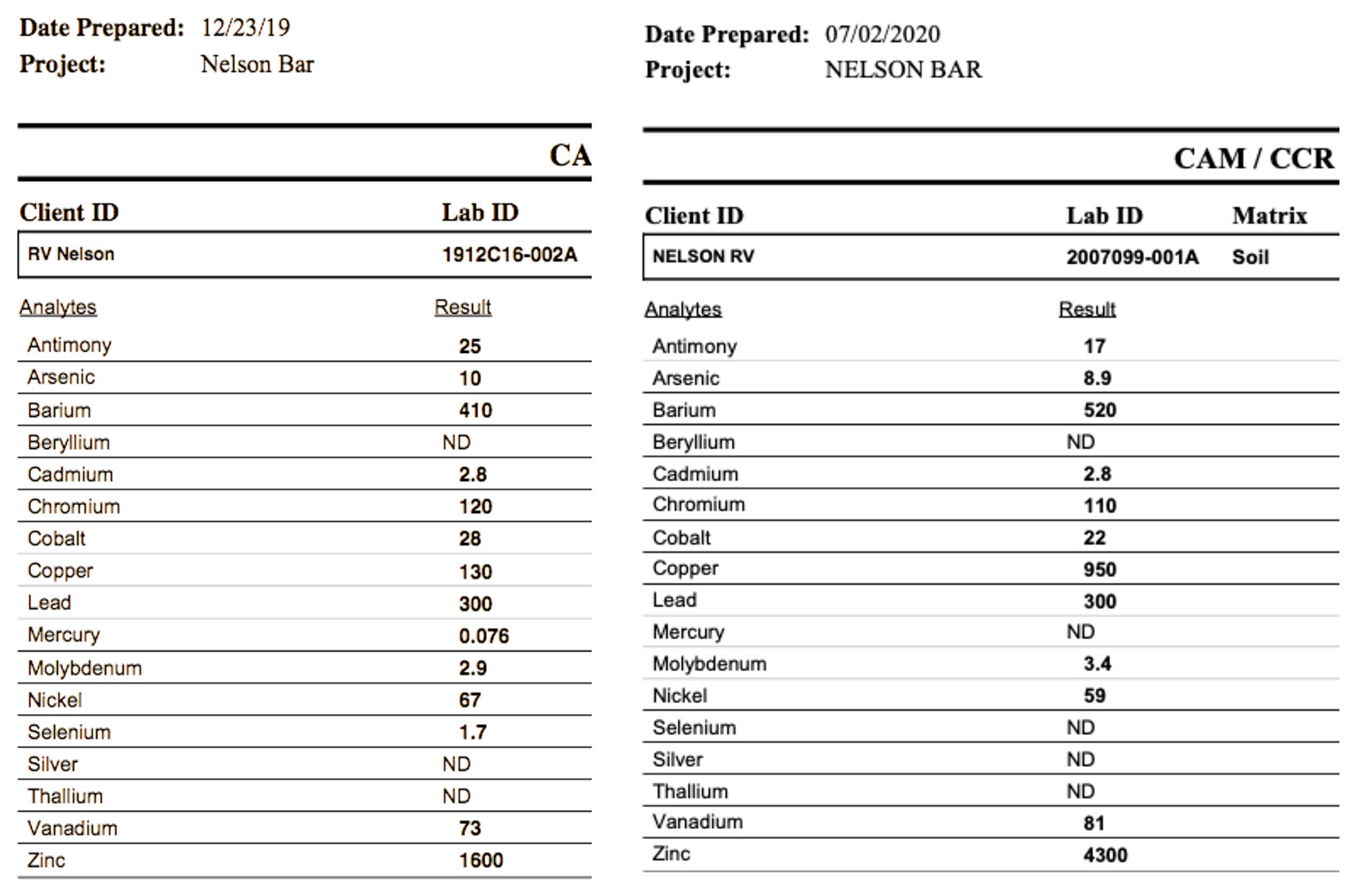
Figure 10. Before (left) and after (right) values for heavy metals reported in mg/kg on a dry weight basis. Very few metals were reduced over the duration of this site treatment and some increased. Most noteworthy were the significant increase in copper (9X) and zinc (nearly 3X). Again this could be attributed to sampling error or increase availability/mineralization due to further degradation of ashen debris. This site was particularly poorly drained and on gravel, possibly creating a soil environment that inhibits good mycelial growth and hence of poor metal uptake.
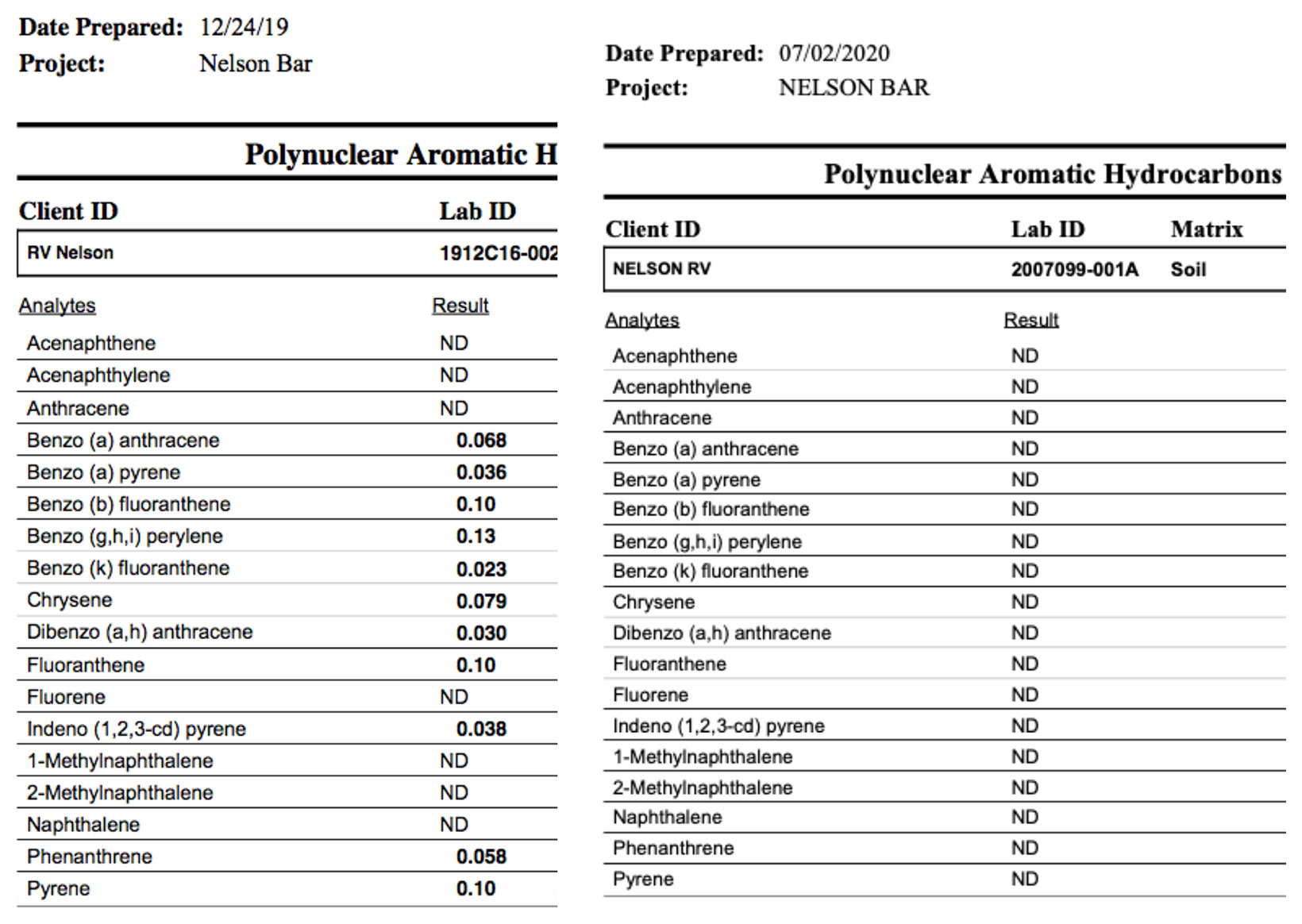
Figure 11. Before (left) and after (right) values for Polycyclic Aromatic Hydrocarbons (PAHs) reported in mg/kg on a dry weight basis. Over the duration of the treatment there was a 100% reduction of PAHs for all types. While reduction of heavy metals, for this site treatment was unsuccessful, the total degradation of PAHs marks a successful reduction of toxicity in the ash footprint.
Conclusions
For each remediation site, soils were sampled for a panel of heavy metals, benzene, dioxin, and polycyclic aromatic hydrocarbon. In most circumstances heavy metals were the primary toxin present. Soil tests performed in this study have demonstrated the presence of environmental pollutants in fire events, including lead, cadmium, chromium, barium, and zinc. Dioxin and PAHs were around on two different respective locations. Taking into consideration that these tests were performed over a year after the fire event, this study demonstrates the concern over environmental pollutants persisting after fire events is very much real.
No benzene detected in any site indicating biodegradation by native microbial populations within the first several month. Still, it is noteworthy that deploying fungal material immediately after fire events may still provide meaningful impact to reducing this pollutant.
Heavy metal uptake by fungi may be the greatest/most effective in the most extreme/highest rates of contamination. Conversely when heavy metal contamination rates were not as high, uptake rates by fungi are typically less pronounced. In addition there were some instances where there was a slight increase in heavy metal in post-treatment samples, this is due to sampling error or further mineralization of metals over time.
In the instances of dioxin and PAHs, there was significant reduction of pollutants after fungal treatment. Deployment of fungal materials may be most helpful directly after a fire even for the biodegradation of persistent organic pollutants and minimization of their movement in water and soil.
As part of the monitoring process for bioremediation sites, the mushroom fruit bodies that sprouted from the sites were sampled. These mushrooms were then dried, homogenized and sent to the lab for heavy metal analysis. The purpose of this was to serve an indicator of heavy metal uptake, but also to determine if any significant amount of heavy metal was being translocated into the mushroom.
While some literature indicates that fungi will translocate heavy metals to the above ground portion of the fungus/mushroom, no significant metal content was observed in the mushrooms sampled.
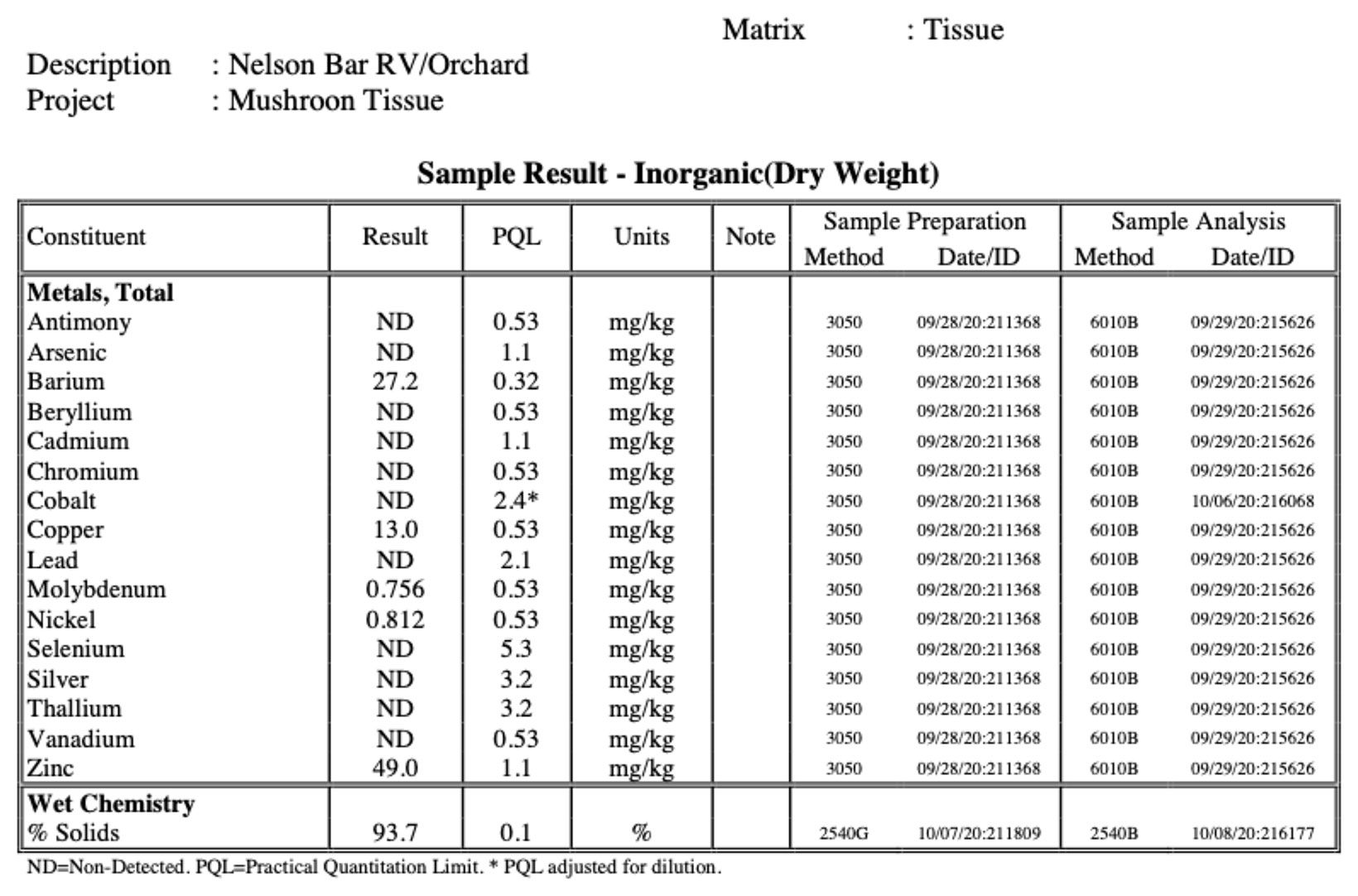
Figure 12. Tissues sample from mushrooms taken from the ash footprint of an RV. This table shows levels of heavy metals translocated into the fruit body reported in mg/kg on a dry weight basis. The copper and zinc levels are comparable to that in commercially produced mushrooms. Normal/Safe barium for mushrooms is unknown to this author.
Due to seasonal and time restrictions Oyster mushroom (Pluerotus ostreatus) was the only fungus applied in this project. While oyster mushrooms are an excellent candidate for biodegradation of persistent organic pollutants, they are limited in which heavy metals they can uptake. By employing species of fungi that are know to pick up specific heavy metals, more effective heavy metal reductions could be achieved. Limitations to this strategy include varieties that are insufficiently aggressive or seasonally/regionally inappropriate and the lack of available mycelium for a rapid response to fire events.
Based on this author's observations one of the best possible options for a rapid response to fire events with mycoremediation techniques would be the utilization of Jute Landscape Fabric. It is inexpensive, easily sterilized with calcium hydroxide, and supports fungal growth well. It is important to have adequate nutrient in bioremediation substrates to adequately support the growth of the fungus, but is not so nutrient rich that the fungus will not grow beyond the substrate into contaminated soil. Soil to ash contact is critical, and Jute fabric has a high surface to volume ratio.
In addition, the woven Jute material can be deployed as a ready-made mycelial mat, twisted into noodles for wattles, or rolled up to make burlap bags. By slightly augmenting this Jute material, which is an industry standard for erosion control, it may be possible to reduce the toxicity of ash-laden soil via the degradation of organic pollutants and immobilization of heavy metals for easier removal.
Wildfires across the world are becoming more frequent, larger in scale, and higher intensity. It is clear that incinerated materials found in human-made products present a risk to humans, plants, animals and the hydrologic cycle at large. Improved methods of dealing with the toxic fallout from these events is critical to the health of the environment. While fungal bioremediation has its limitations in ameliorating toxic material, it can be part of the solution. By incorporating fungal biology into the Best Management Practices employed in the wake of a fire event we may be able to better immobilize toxins and reduce the spread of pollutants.
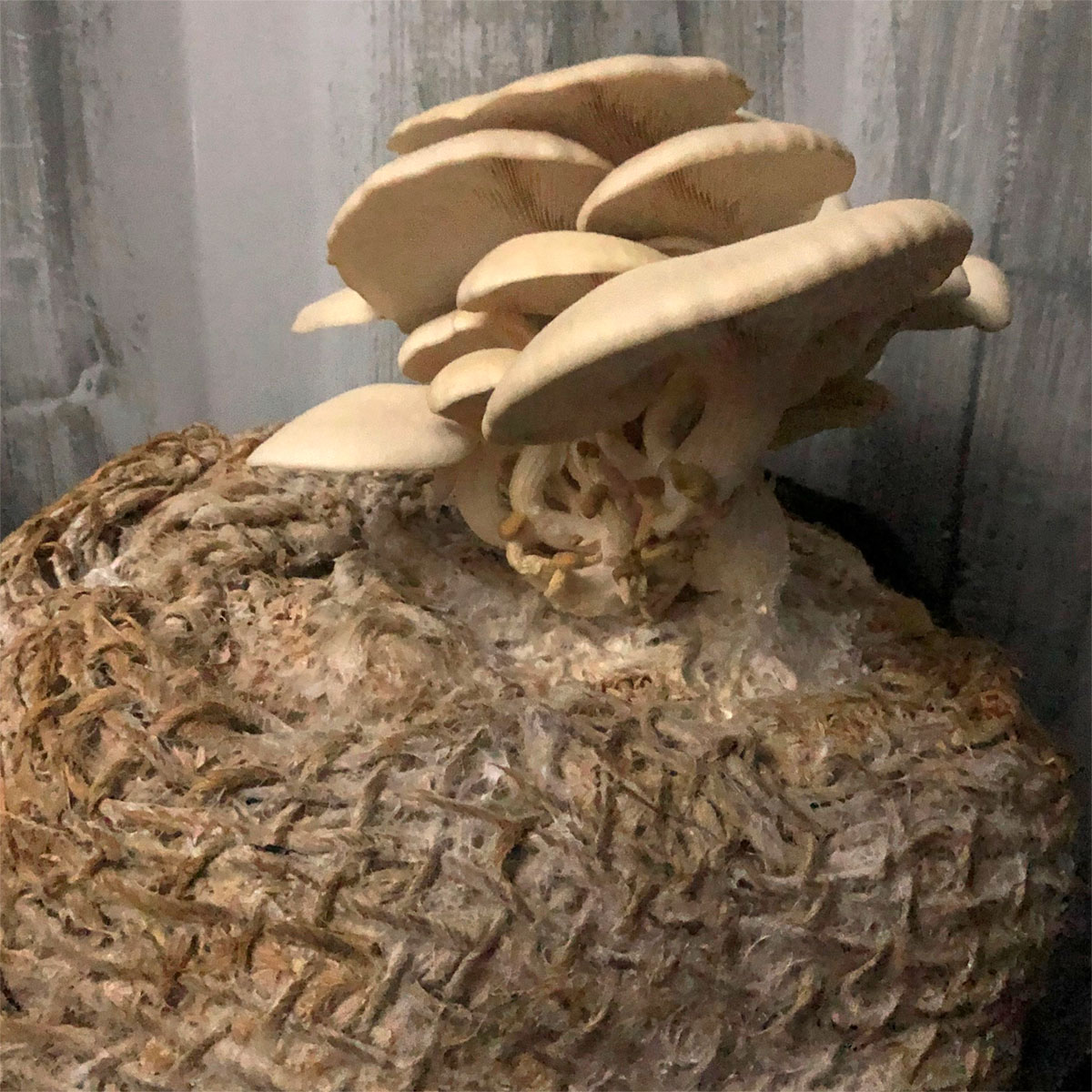
Figure 13. Roll of Jute Landscape Fabric fully colonized by Oyster mushroom mycelium, with mushroom sprouting from it.