Fire Borne Toxin Remediation On-Farm
In November of 2018 the Camp Fire burned through TurkeyTail Farm, destroying buildings and vehicles. Due to the scale of the disaster FEMA declared all the burn scar as a natural disaster site, and required regulatory oversight of the clean-up.
While much of the debris was removed and soils were tested to ensure heavy metals were below an acceptable level, clean-up structures smaller than 120 square feet and vehicles went overlooked from this sampling. In the circumstance of TurkeyTail Farm several cars and a shed were not addressed.
Fire born toxins and debris have long lasting consequences for farm ecology. While the debris was removed, the ash from burned structures and vehicles was driven into the soil by heavy machinery traffic and heavy rains. Short of removing all of the top soil, removal of toxic ash is impossible. The fire baked soils become hydrophobic and due to contamination, revegetation is slow to occur.
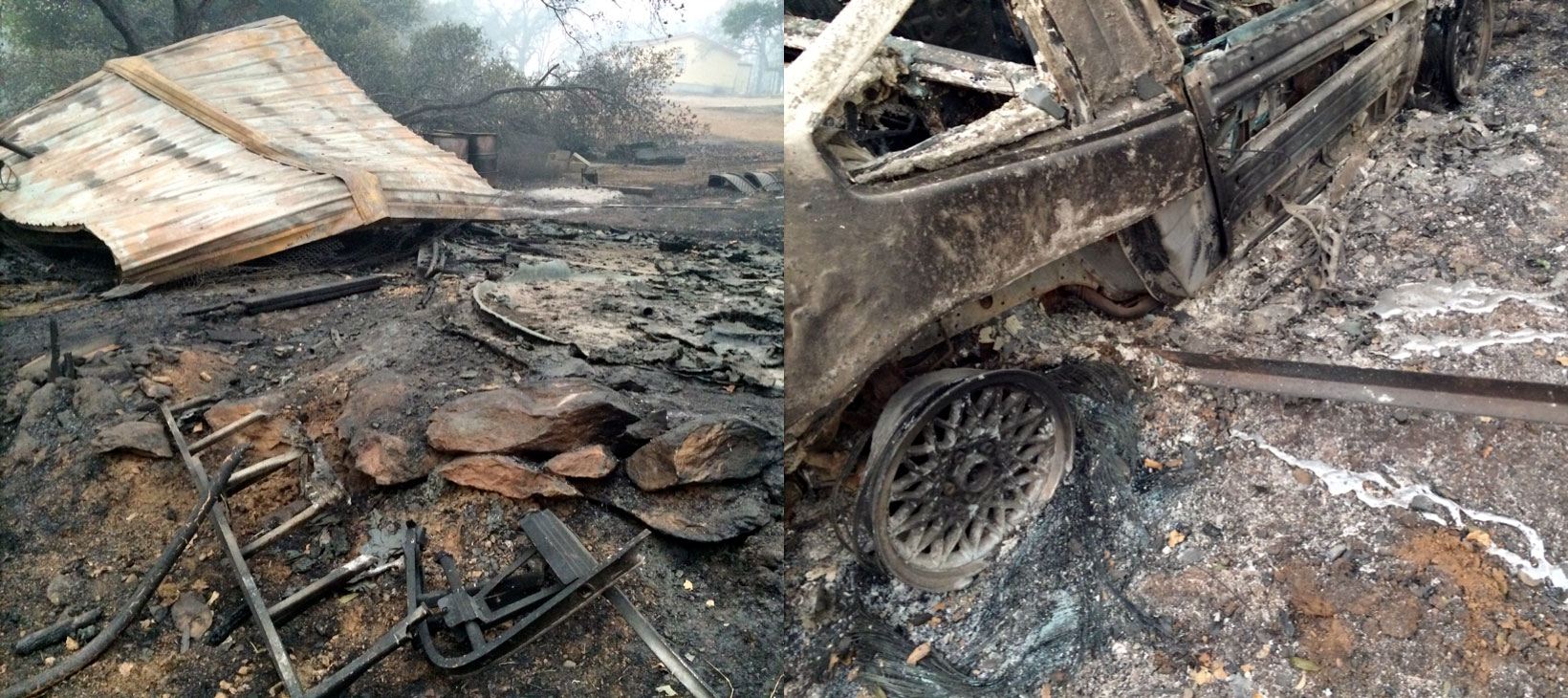
Figure 1. Burned structures and vehicles located at TurkeyTail Farm.
Two different locations were identified as potential sources of contamination, one being the location where several vehicles burned and the other being a site where a shed had burned. For each site a 20’ × 20’ plot was identified. Samples were taken from three separate locations for each plot respectively and homogenized. Sample sites were marked with landscape flags so the precise location could be resampled at a later date. Homogenized samples from these two respective sites were sent to the lab for a panel of toxins that are associated with fire events: dioxins, benzene, polycyclic aromatic hydrocarbons, polychlorinated biphenyls, and heavy metals. The “CAM 17” is the standard test used in the FEMA debris removal program. In addition to these 17 target heavy metals, aluminum was also tested for as it is an overlooked potential contaminate.
Of all the pollutants tested for, only heavy metals were detected.
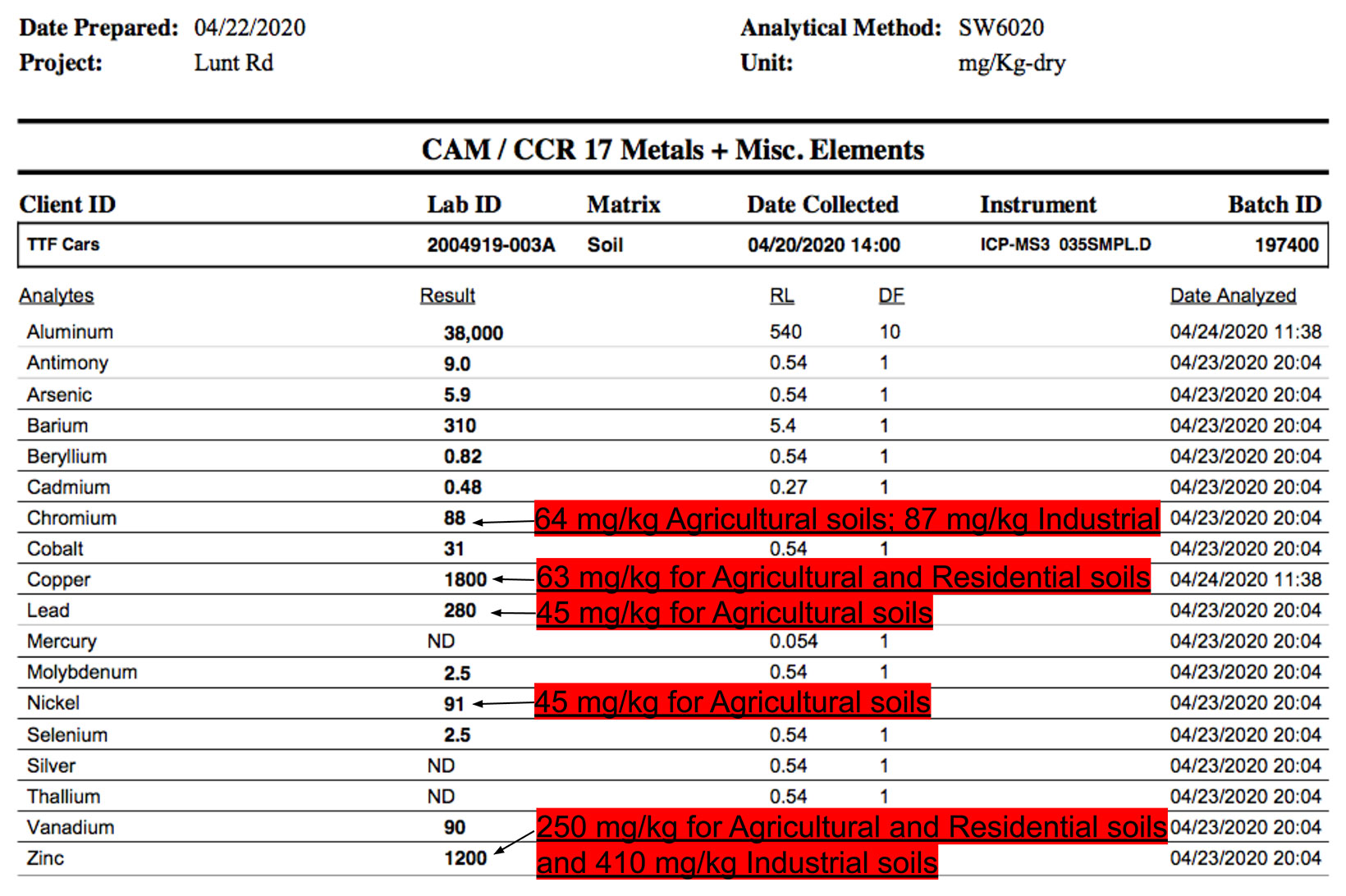
Figure 2. Heavy metal values for the burned cars' ash footprint reported on the basis of mg/kg on dry weight basis. Red highlights have the threshold for safe and allowable levels based on Canadian Council of Ministers of the Environment standards. Most notable values above standard are chromium, copper, lead, nickel, and zinc. While the Canadian Council does not have a standard for aluminum, levels of soluble Al+++ above 1 mg/kg can negatively impact plant growth.
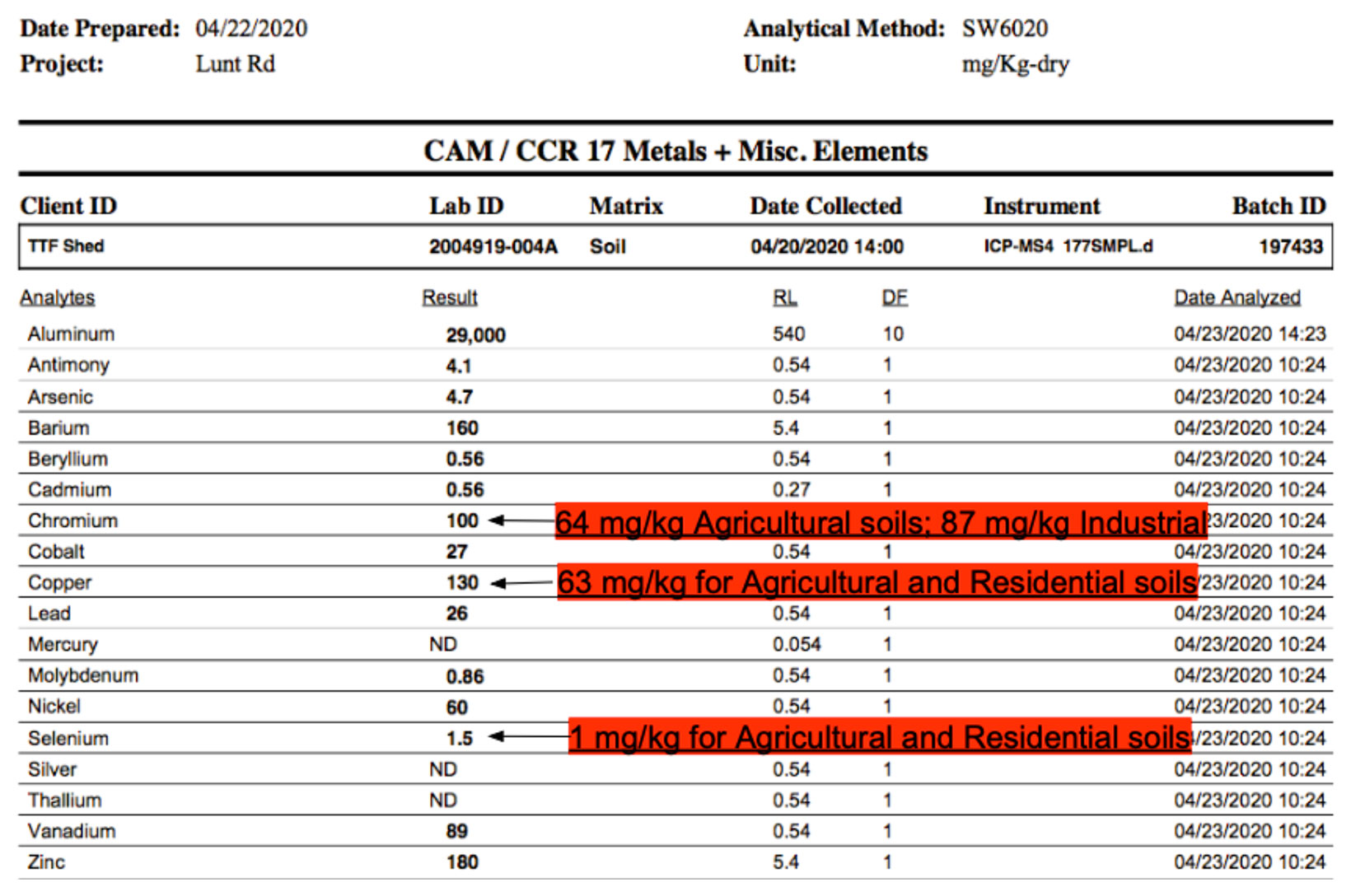
Figure 3. Heavy metal values for the ash footprint of burned cars reported on the basis of mg/kg on dry weight basis. Red highlights have the threshold for safe and allowable levels based on Canadian Council of Ministers of the Environment standards. While the Canadian Council does not have a standard for Aluminum, levels of soluble Al+++ above 1 mg/kg can negatively impact plant growth.
Oyster mushroom (Pleurotus ostreatus) mycelium grown on rice straw served as a source of fungal inoculum (termed spawn). To grow oyster mushroom spawn, rice straw is pasteurized in 180 degree Fahrenheit water, drained, then allowed to cool. The straw is inoculated at the rate of 1 part myceliated wood chip spawn to 10 parts pasteurized straw, and then placed in clean bags to incubate for two weeks. After a period of two weeks the fungus completely colonizes to straw and is ready to apply to the field.
Approximately 1 cubic yard of mushroom spawn was used to inoculate each 20’ × 20‘ plot. To maximize soil-mushroom contact, the 20’ × 20’ plots were rototilled lightly to loosen the soil surface, the mushroom spawn was shredded across the plot, and tilled again to integrate mushroom matter and maximize mycelial contact. The plot was then covered with oyster mushroom impregnated jute landscape fabric for moisture retention and additional mycelial mass. Finally the plot was mulched with additional rice straw to protect mushroom spawn and retain moisture. Overhead irrigation was applied as needed to sustain the fungus.
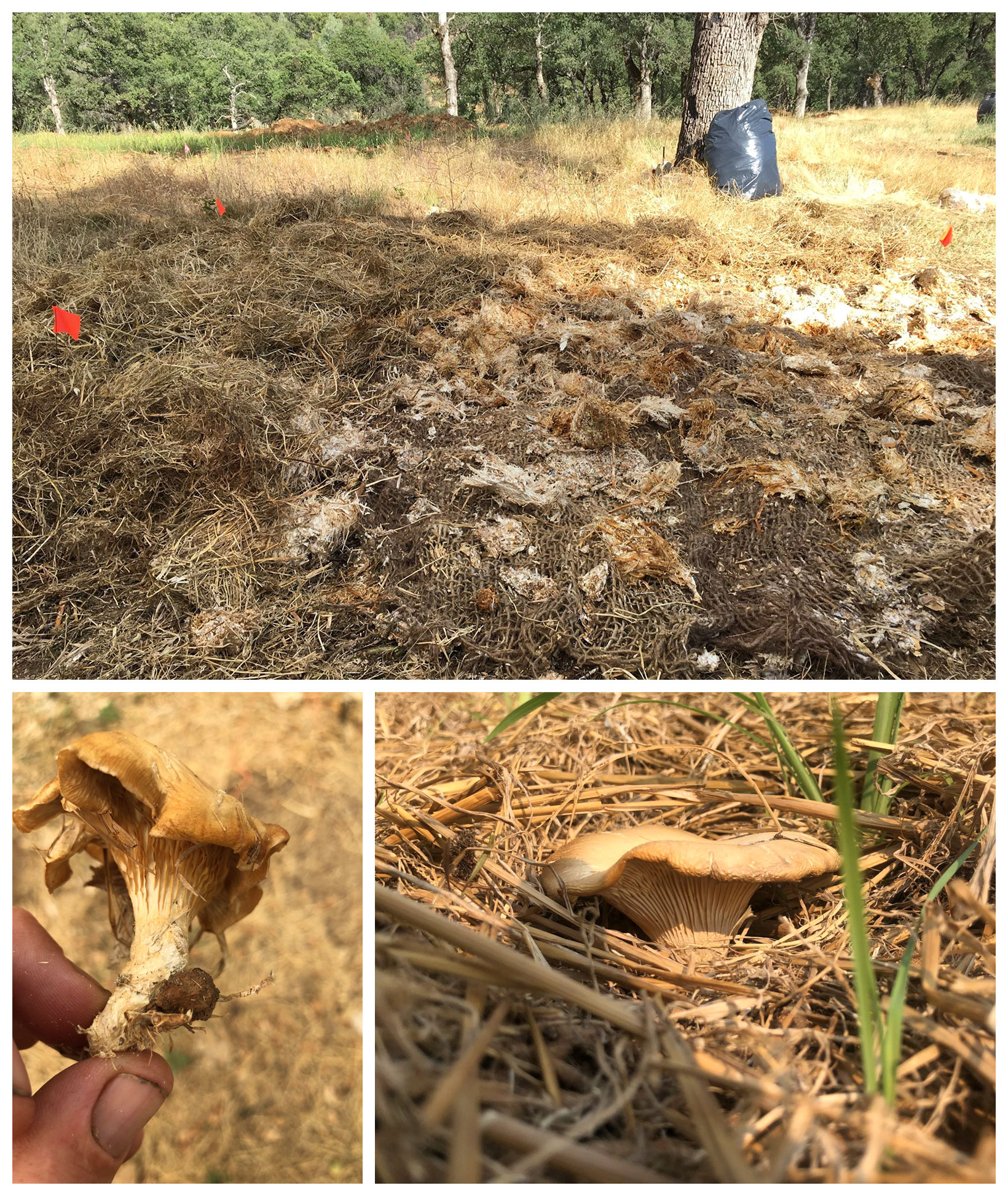
Figure 4. Top: Application mushroom spawn to contaminated plot. Bottom: Mushrooms sprouting from treatment plots.
Over the duration of the treatment, mushrooms produced out of the plot were sampled and dried. After a sample size big enough was accumulated, mushrooms were submitted to the lab. After a duration of 6 months sites were resampled.
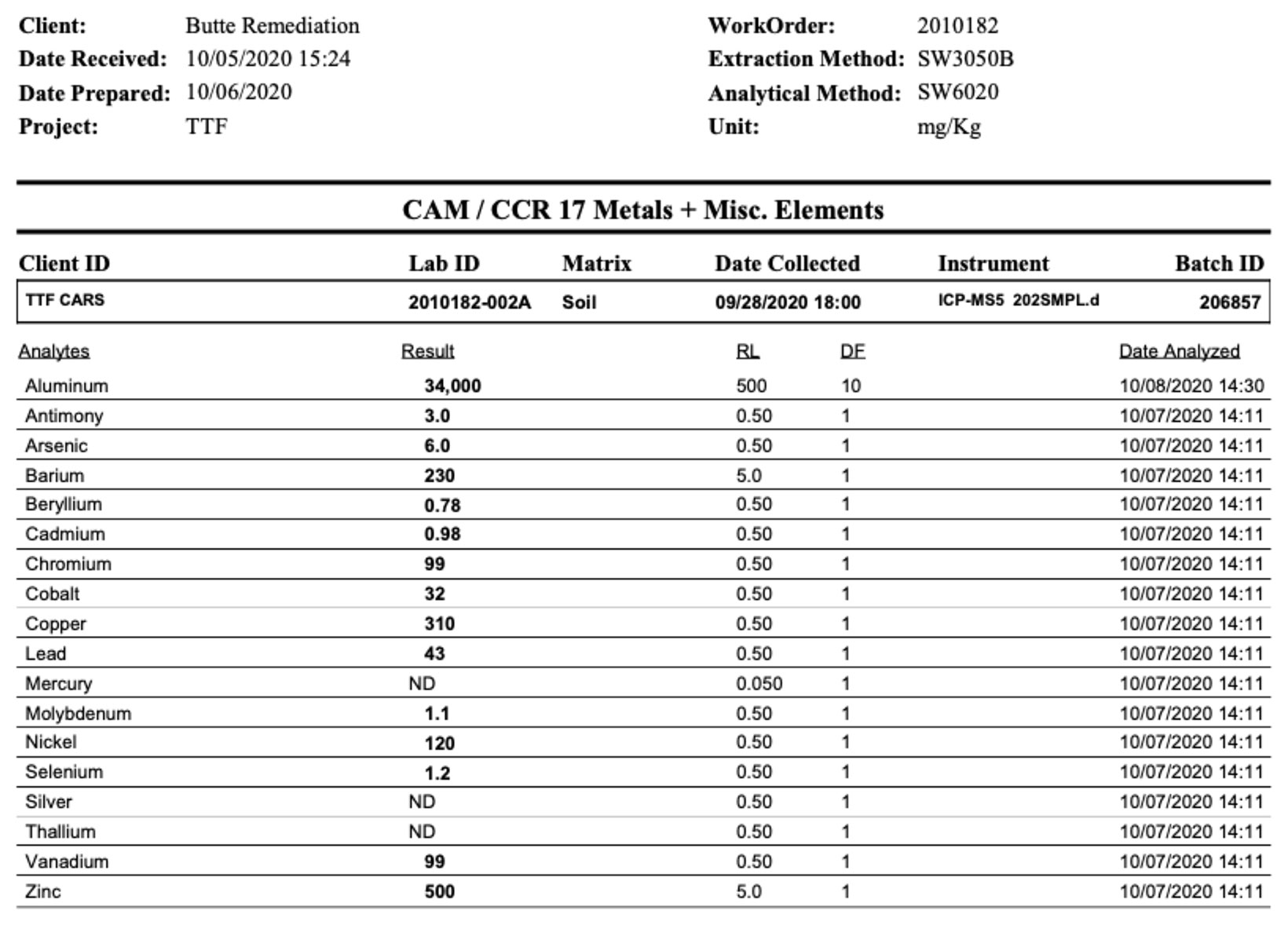
Figure 5. Heavy metal values for the burned cars ash footprint after fungal treatment reported on the basis of mg/kg on dry weight basis.
Not all target heavy metals were successfully reduced, but there were significant reductions in copper (from 1800 to 310 mg/kg), lead (from 280 to 43 mg/kg), and zinc (from 1200 to 500 mg/kg).
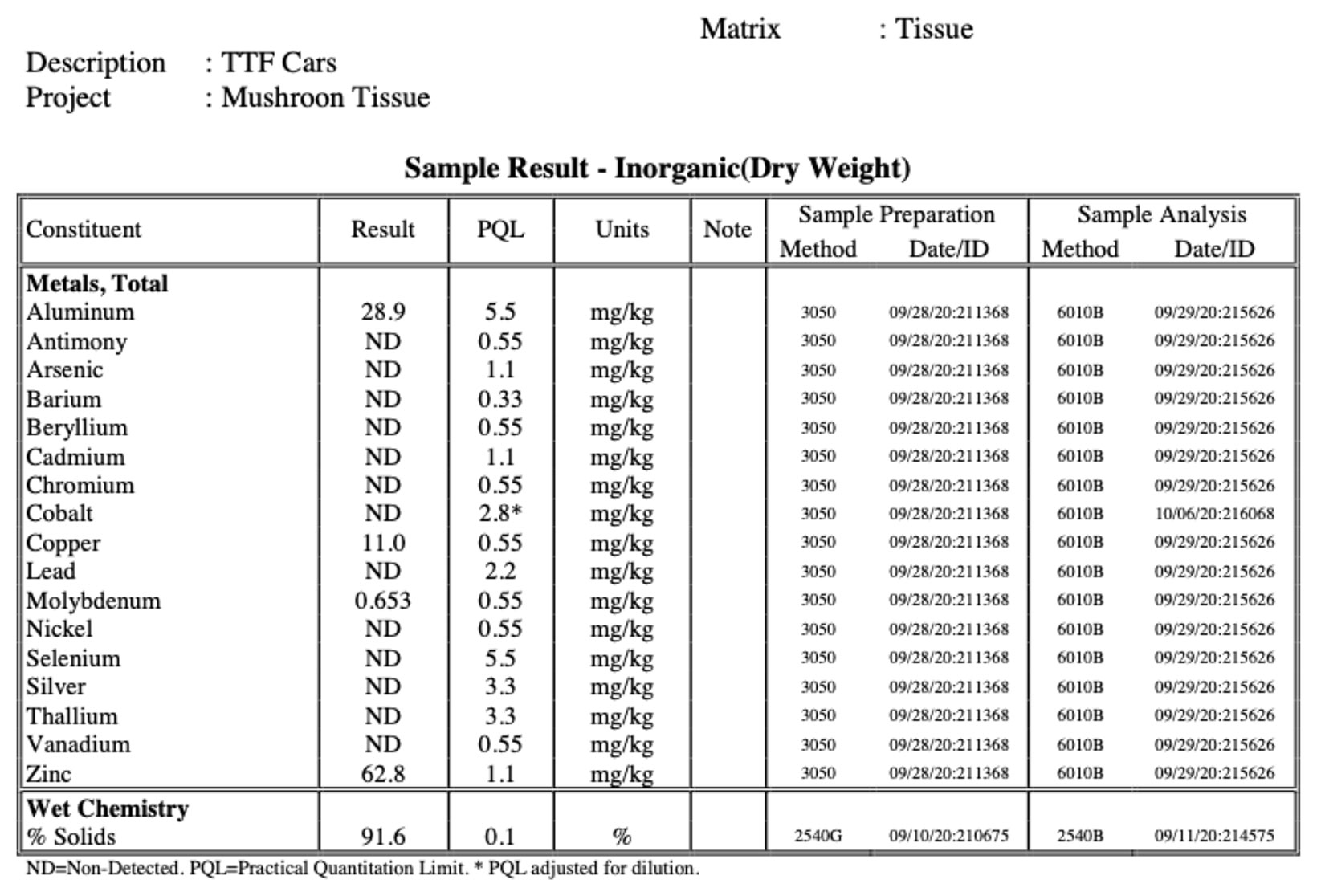
Figure 6. Tissues sample from ash footprint of burned cars. Analysis of mushrooms showing the levels of heavy metals translocated into the mushroom itself reported in mg/kg on a dry weight basis. All the metal levels are within the normal range for mushrooms, indicating no significant translocation of metals into the mushroom fruit body.
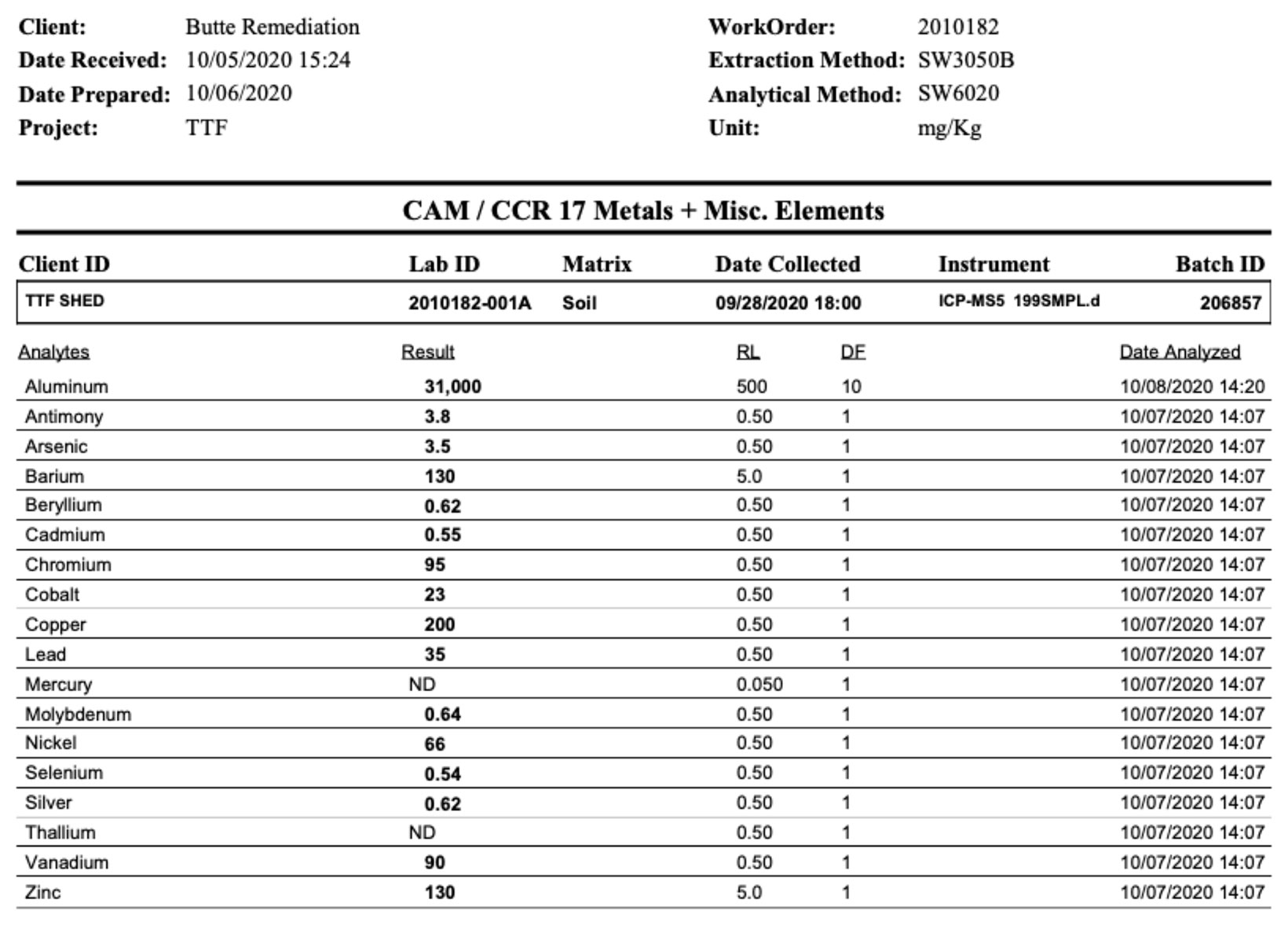
Figure 7. Heavy metal values for the shed ash footprint after fungal treatment reported on the basis of mg/kg on dry weight basis.
In the circumstance of the shed site there was no significant reductions of heavy metals. In a few instances there was a slight increase in metal values, specifically aluminum (from 29,000 mg/kg to 31,000 mg/kg) and copper (from 130 mg/kg to 200 mg/kg). There are several possible explanations for this including sampling error or continued mineralization of toxic metal via continued breakdown of toxic materials.
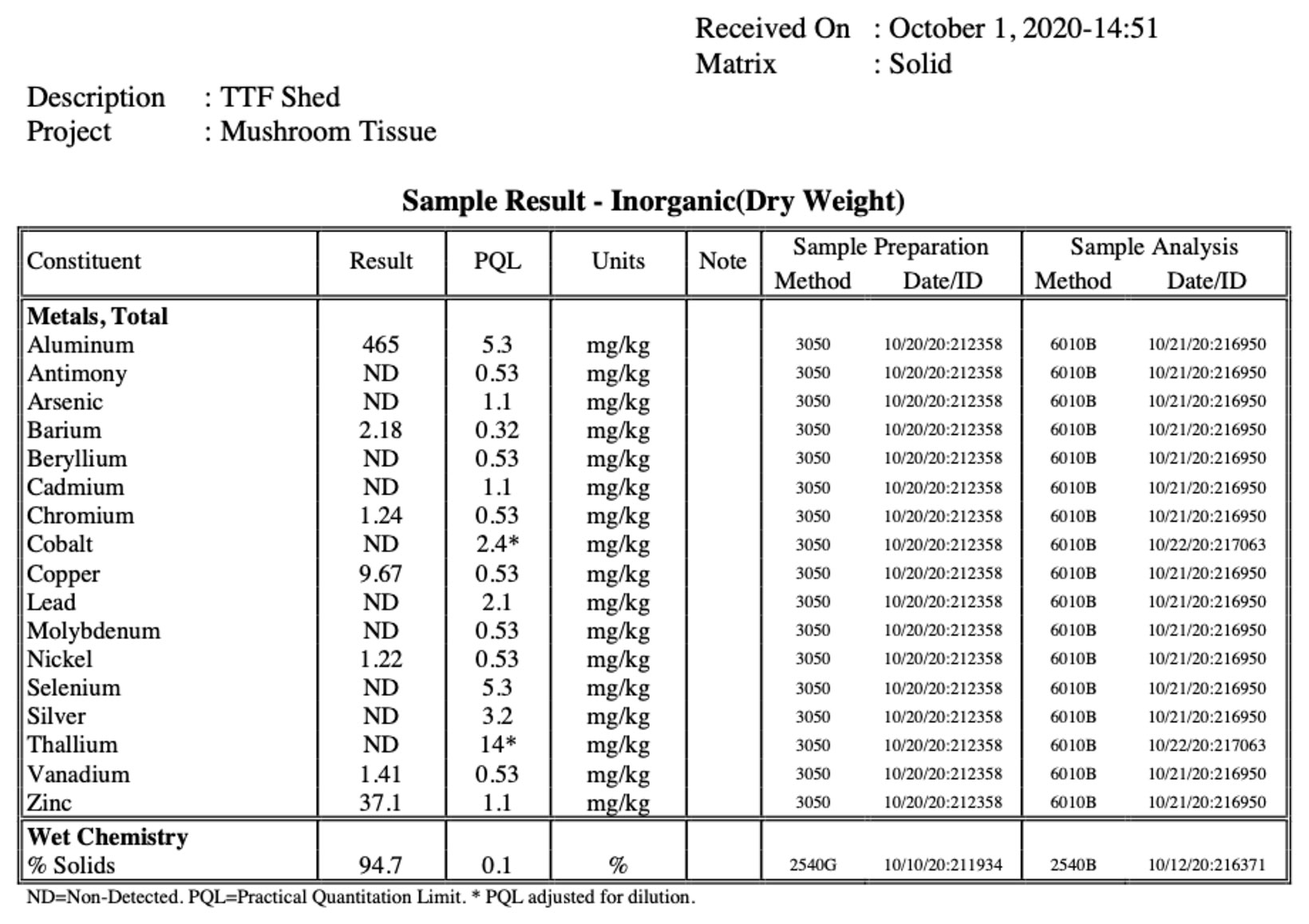
Figure 8. Tissues sample from ash footprint of shed. Analysis of mushrooms showing the levels of heavy metals translocated into the mushroom itself reported in mg/kg on a dry weight basis. The copper and zinc levels are comparable to that in commercially produced mushrooms. Normal/Safe barium for mushrooms is unknown to this author. Most notably there was a significant amount of Aluminum found in mushroom fruit bodies. Aluminum content is 18 times that of mushrooms found in the wild (465 mg/kg verses 10-25 mg/kg in wild mushrooms).
Conclusions
For each remediation site, soils were sampled for a panel of heavy metals, dioxins, benzene, polycyclic aromatic hydrocarbons, and polychlorinated biphenyls. Heavy metals were the only environmental contaminant present. Taking into consideration that these tests were performed over a two years after the fire event, this study demonstrates the concern over environmental pollutants persisting for long periods after fire events is very much real.
The ash footprint from the vehicles showed levels of contamination above safe and allowable standards for chromium, copper, lead, nickel, and zinc (based on Canadian Council of Ministers of the Environment standards). The ash footprint of the shed demonstrated much lower contamination levels. For the shed site, chromium and copper were above safe and allowable standards, and only marginally in excess.
In initial soil samples aluminum values were between 29,000 mg/kg and 38,000 mg/kg. While the solubility of this aluminum is unclear, it was confirmed with the lab that performed the analytics that this was exchangeable aluminum (as opposed to the aluminum that make up of the core of crystalline lattice of clay soil). It is common for fire exposed aluminum to be pushed from its Al++ to the trivalent Al+++ in fire events. While the Canadian Council does not have a standard for total aluminum, levels soluble Al+++ above 1 mg/kg can negatively impact plant growth. The presence of aluminum coupled with excessively high copper concentrations my be part of the reason revegetation after fire events is slow.
No persistent organic pollutants (benzene, dioxin, PAHs, PCBs) were observed. There could be several reasons for this; the pollutants may have been removed with the then debris, already precipitated into soil strata, or naturally degraded over the two years since the fire event.
Mushroom installations were successfully implemented, showing mycelial growth throughout the project. Mycelium showed active growth into contaminated soil and aggregated soil particles. Mushroom fruit bodies were successfully sprouted from remediation sites.
The ash footprint of the cars showed some successful reduction in heavy metal values over the span of the study with significant reductions in copper (5X reduction), lead (6X reduction), and zinc (2X reduction). The ash footprint of the shed had much lower initial contamination rates and virtually no reduction in heavy metal levels over the duration over the study.
The initial remediation trials immediately after the Camp Fire have shown that fungal treatments are most effective and show the greatest rates of reduction when the contamination rates are the highest/most severe. Conversely, when contamination rates are less pronounced, fungal remediation seem to be less effective. The rates of reduction in this study seem to be in line with that observation.
As part of the monitoring process for the two sites, the mushroom fruit bodies that sprouted from the sites were sampled. These mushrooms were then dried, homogenized and sent to the lab for heavy metal analysis. The purpose of this was to serve an indicator of heavy metal uptake, but also to determine if any significant amount of heavy metal was being translocated into the mushroom.
While some literature indicates that fungi will translocate heavy metals to the above ground portion of the fungus/mushroom, in initial trials no significant metal content was observed in the mushrooms sampled. In this study the ash footprint of the shed did show a spike of aluminum in the mushroom fruit bodies that were sampled. In the composite sample aluminum content is 18 times that of mushrooms found in the wild (465 mg/kg verses 10-25 mg/kg in wild mushrooms). Interestingly the aluminum values in that plot soil increased over the duration of the study from 29,000 mg/kg to 31,000 mg/kg. So while the aluminum content of mushroom tissue sample implies the functional uptake of Al molecules, and that there may also continued mineralization of pollutants.
It is this authors conjectures that possibly the generation organic acid in biological degradation of organic matter and continued weathering of soils may release more toxic materials into a soluble state over time. Alternatively, this increase in aluminum content could be explained by sampling error. While considerable care was taken to ensure the exact same location was tested for follow-up samples, it is possible that a portion of soil especially high in aluminum was incorporated into the sample.
Wildfires across the world are becoming more frequent, larger in scale, and higher intensity. It is clear that incinerated materials found in human-made products present a risk to humans, plants, animals and the hydrologic cycle at large. Improved methods of dealing with the toxic fallout from these events is critical to the health of the environment. While fungal bioremediation has its limitations in ameliorating toxic material, it can be part of the solution. By incorporating fungal biology into the Best Management Practices employed in the wake of a fire event we may be able to better immobilize toxins and reduce the spread of pollutants.