Degradation of Pesticide Residues On-Farm Using Fungi
The purpose of this study is to examine the ability of fungi to degrade pesticide residues in soil using the enzymatic capabilities of fungi. Many en-vitro studies have demonstrated the ability of fungi to degrade a wide array of pesticides including organophosphates, chlorinated compounds and DDT. The purpose of this study is to evaluate the effectiveness of fungi to degrade pesticides in real-world/en-vivo conditions.
This model farm in this study is a walnut orchard transitioning to organic. This walnut orchard has an 80 year history of conventional (pesticide/herbicide based) management and, under new ownership, is in the process of converting to organic vegetable production.
Initial Soil Testing
The first step in the process was to identify what pesticide residues may be present. Historical pesticide use was determined by pulling the spray records of the previous 2019 year from the records of the regional Ag Commissioner. According to these records the previous years applications included several broad spectrum herbicides.
These included paraquat dichloride (Gramoxone herbicide), acetamiprid (Assail insecticide), sethoxydim (Poast herbicide) and saflufenacil (Treevix herbicide). In addition, by personal conversation with the previous farm manager it was discovered that additional herbicide application occurred, not included in the spray records. It was learned that annual applications of glyphosate (Roundup herbicide) and glufosinate (Liberty herbicide) had also been applied to the orchard in the previous year.
To determine which pesticides residues had persisted into the 2020 year, a sample plot was selected to test for the full panel of know pesticide applications. Samples were taken to the depth of 5”, in three sample locations, then homogenized prior to being submitted for laboratory testing. Labs were unable to test for paraquat dichloride due to its volatility, likewise was glufosinate was not an available test. In addition for testing for glyphosate, samples were also tested for aminomethylphosphonic acid (AMPA) one of the primary degradation products of the glyphosate.
Of the materials tested for, only glyphosate and AMPA were present.
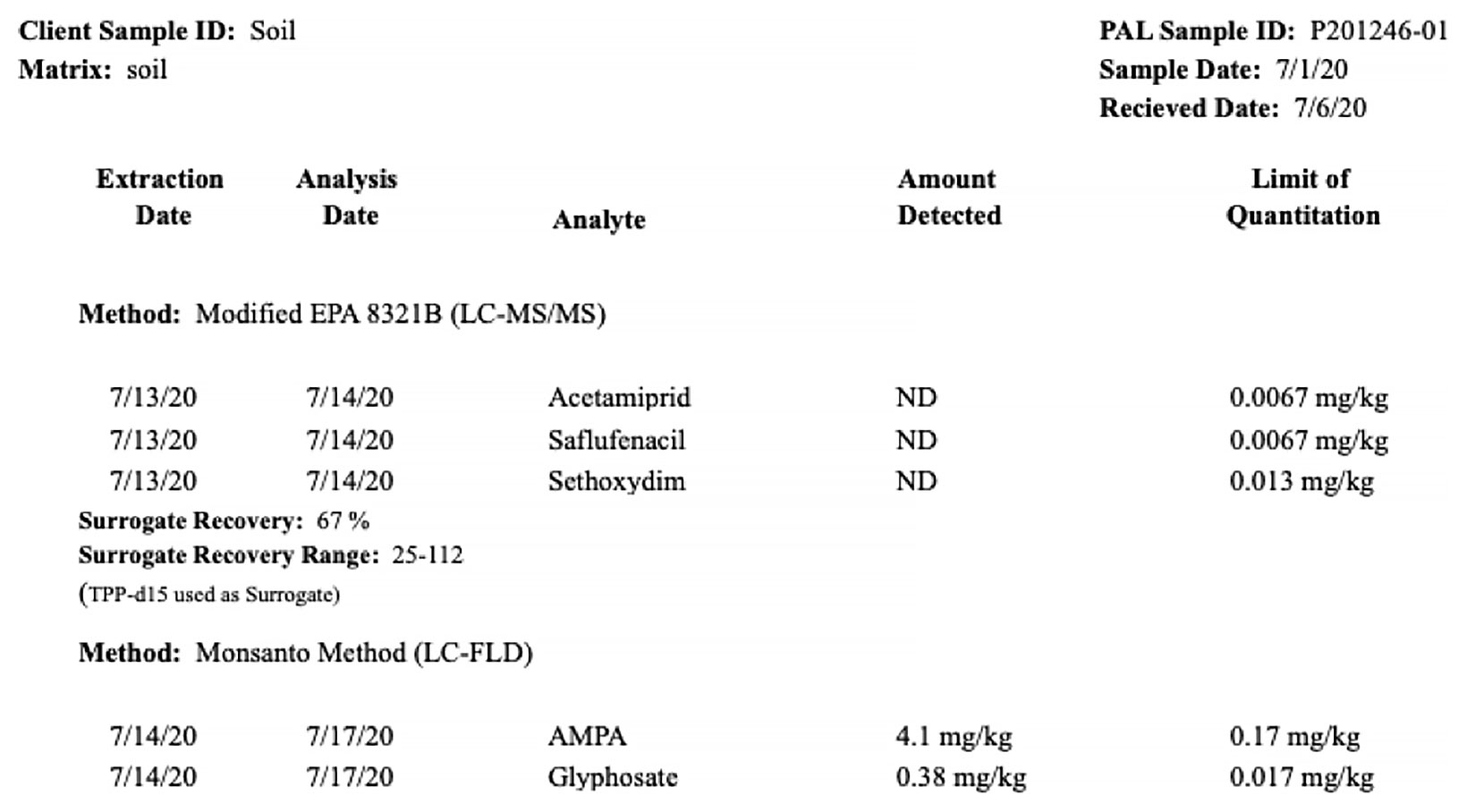
Figure 1. Results from initial sample site to determine the presence of pesticide residues reported in mg/kg on a dry weight basis. Only glyphosate and AMPA were detected.
Glyphosate and AMPA
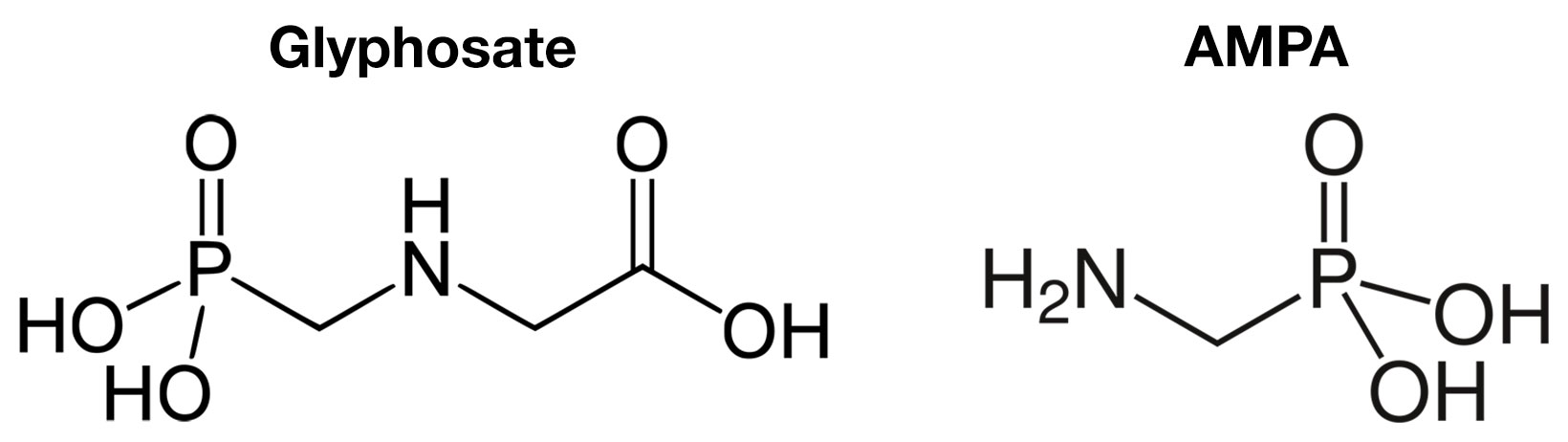
This glyphosate/glufosinate blend of pesticides is a somewhat commonplace practice in orchard management. While their spectrum of control is comparable for several weed species, glufosinate tends to be more effective on annual broadleaf weeds than annual grasses, while glyphosate is more effective on monocots/grasses. Glufosinate is a "contact" herbicide, in contrast to glyphosate being extensively translocated within the plant.
Studies on examining the chemical half-life of glyphosate are controversial, with reported lifespan of anywhere from 2-200 days. It is widely understood that glyphosate has an affinity to bind to soil particles and thus mostly accumulates in the top-soil layers. With regular applications there is an average concentration of 1-4 mg/kg in the top 15 cm of soil are not uncommon.
Owing to extensive usage, this chemical may pose chronic and remote hazards to the ecological environment. Research indicates glyphosate may reduce the uptake and translocation of micronutrients in plants. These poorly soluble complexes of glyphosate chelate with micronutrients hinder their root uptake and translocation by crops.
While glyphosate is meant to be a foliar application with uptake through the stomata, glyphosate can be released into the soil as root exudates. In addition, studies have shown glyphosate negatively effects soil biota, inhibiting the growth of bacterial. Recent studies have also shown linkage between glyphosate exposure in humans and negative health effects. Contemporary research shows some connection to endocrine disruption, birth defects and various cancers.
Aminomethylphosphonic acid (AMPA) is a weak organic acid with a phosphonic acid group. It is one of the primary degradation products of the herbicide glyphosate. AMPA has toxicity which is comparable to that of glyphosate and it is therefore considered to be of similar toxicological concern as glyphosate itself (harmful in greater than 0.5 parts per billion).
Experimental Design and Field Layout
The section of field dedicated to the study was separated into 6 different 10’ × 25’ plots. Each plot was sampled in three separate locations at the depth of 5”, the sample sites were flagged for resampling at a later date, and the samples from each respective plot were homogenized and submitted to the lab for glyphosate and AMPA analysis.
For the purpose of this study 3 of the plots received a fungal inoculation, and seeded with cover crop, and 3 plots served as controls. Each 10’ × 25’ plot was assigned a number 1-6, and treatments were assigned at random.
For each Fungal Treatment Plot received 0.5 cubic yard (14 cubic feet) of rice straw/rice hull oyster mushroom spawn (mushroom impregnated substrate). This mushroom spawn was rototilled into the top several inches of soil, and seeded with cover crop (vetch, clover, faba, wheat).
The Control Plots received one of the three following treatments respectively: application of 0.5 cu.ft. of uninnoculated rice straw/hulls and seeded with cover crop (termed “mulched” for the purposes of this study), cover crop only, and no treatment/no irrigation/no mulch. Overhead irrigation was applied to all of the plots over the duration of the study sufficient to sustain cover crop, with the exception of the “no treatment” plot which was not rototilled, left as bare soil, and no irrigation was applied.
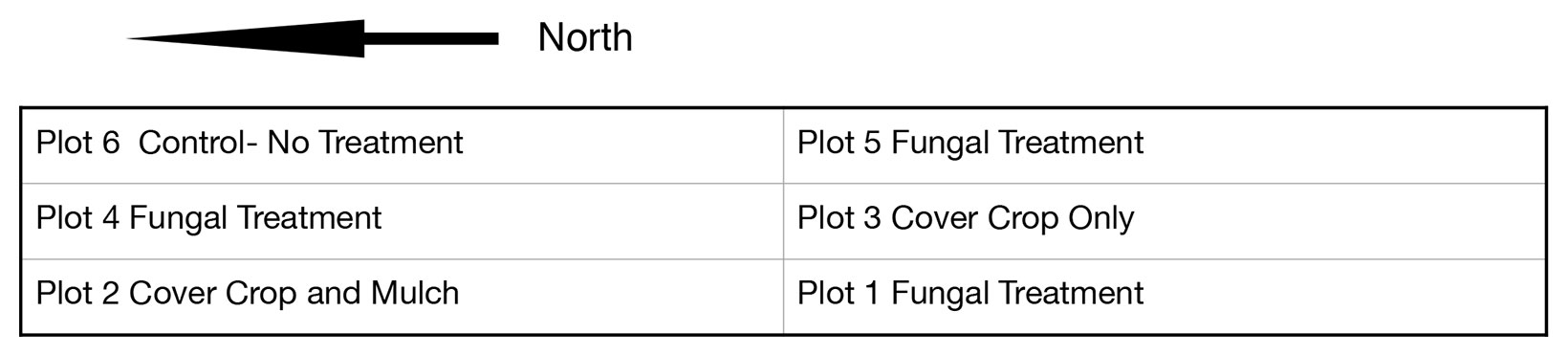
Figure 2. Field Layout of Experiment
Over the duration of the experiment, fungi continued to fruit (produce mushrooms) in Fungal Treatment Plots indicating persistence of mycelium in the soil. In addition, mycelial threads could be observed in the upper strata of the soil.
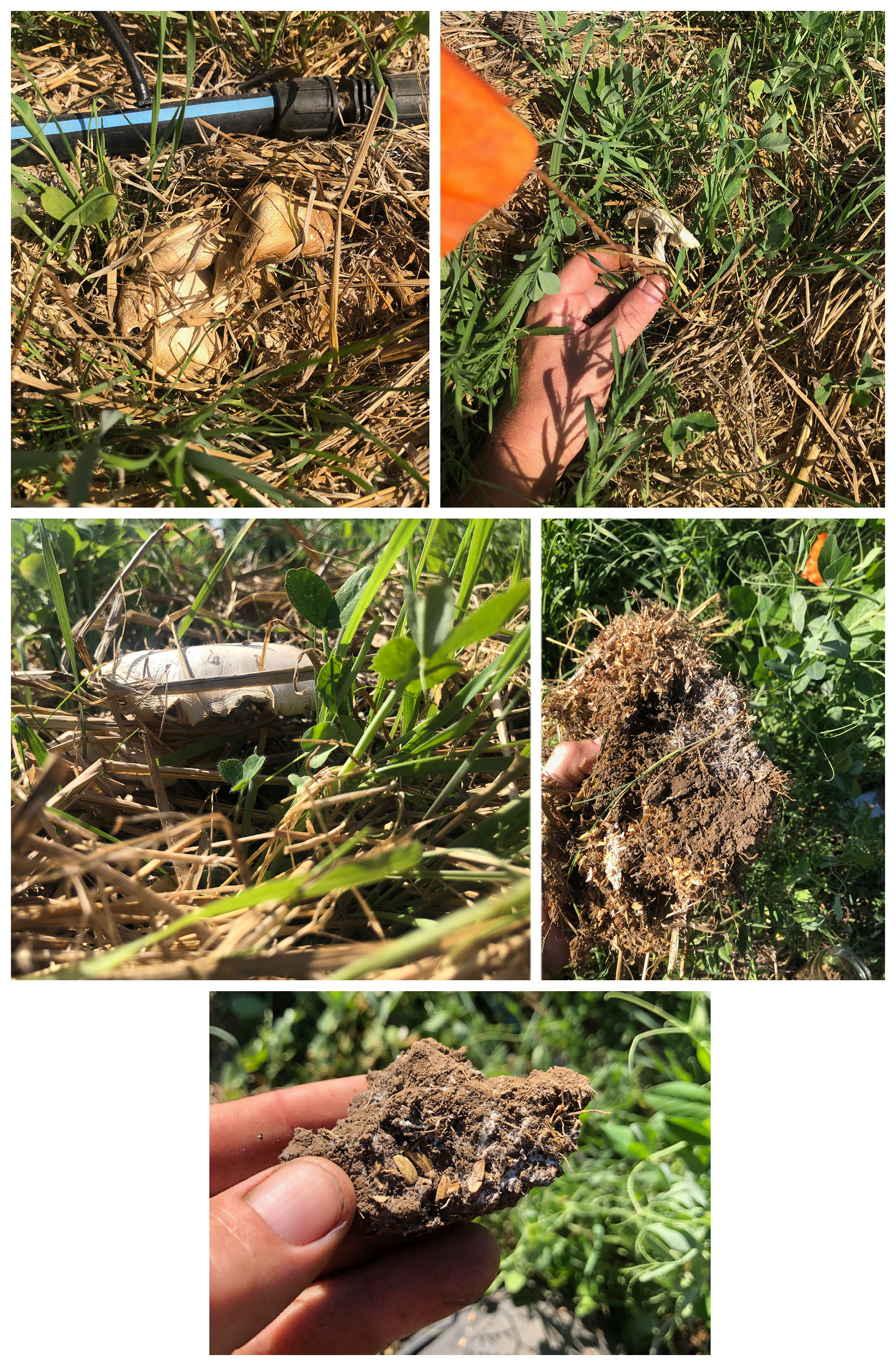
Figure 3. Mushrooms sprouting from Fungal Treatment areas (above and left) and active mycelial growth in soils (below).
After the period of 60 days, the cover crop began to flower; the point in time at which cover crops are typically mowed/tilled-in. At this time the soil was resampled. The original 3 sample sites per plot were identified, sampled to the depth of 5” and homogenized prior to be submitted to the lab for analysis.
Results
Initial, pre-treatment, glyphosate rates ranged from 0.38 mg/kg to 0.089 mg/kg. Initial AMPA rates were significantly higher, ranging from 4.1 mg/kg to 1.4 mg/kg. Over the duration of the study there was a reduction of glyphosate and AMPA in all plots, in some circumstances values being reduced by over half. In the instance of glyphosate, final rates varied from 0.11 mg/kg to 0.036 mg/kg. Values for AMPA ranged from 2.0 mg/kg to 0.92 mg/kg. The greatest overall reduction of glyphosate occurred in Control Plot 6 where no treatment was applied (0.216 mg/kg reduction). In AMPA greatest observed reduction was in Fungal Treatment Plot 1 (2.1 mg/kg).
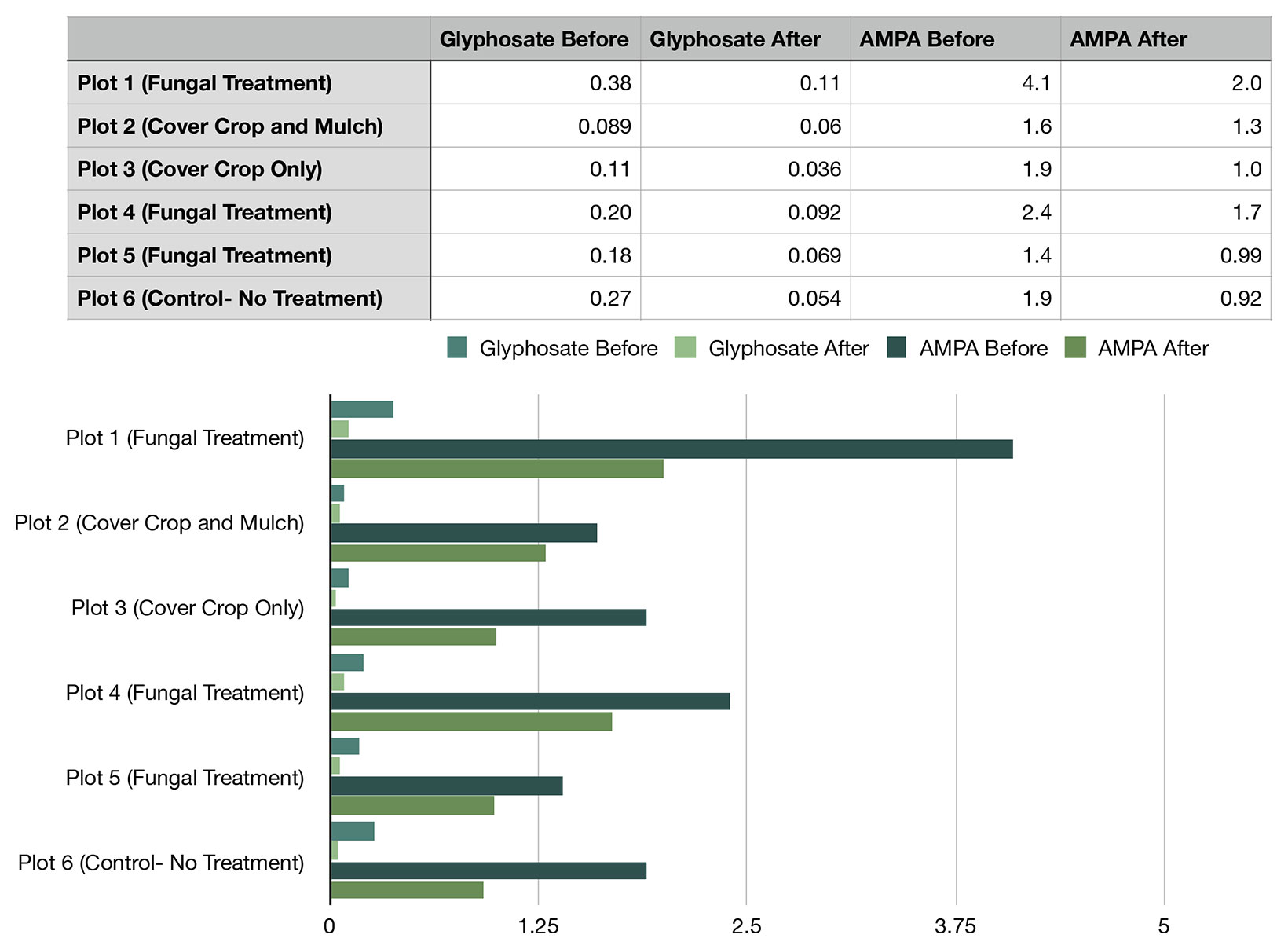
Figure 4. Glyphosate and AMPA values reported in mg/kg dry weight.
With variable pesticide rate across all 6 plots in initial samples, the total rate of reduction does not provide clear indication of effectiveness for the treatments. Evaluation on the basis of percentage reduction from initial to final sample, provides a more proportionate perspective on the rate of reduction over time.
When evaluated on the basis percentage reduction over time, the greatest reduction of glyphosate occurred in Control Plot 6 (no treatment) with an 80% reduction. The lowest rate of reduction was in Control Plot 2 (cover crop and rice straw/hulls mulch) with a 33% reduction. The average rate of reduction across all fungal treatments for glyphosate (plot 1,4, and 5) was 62% (standard deviation of 9%), whereas the average for controls (plot 2, 3, and 6) was 60% (standard deviation of 24%).
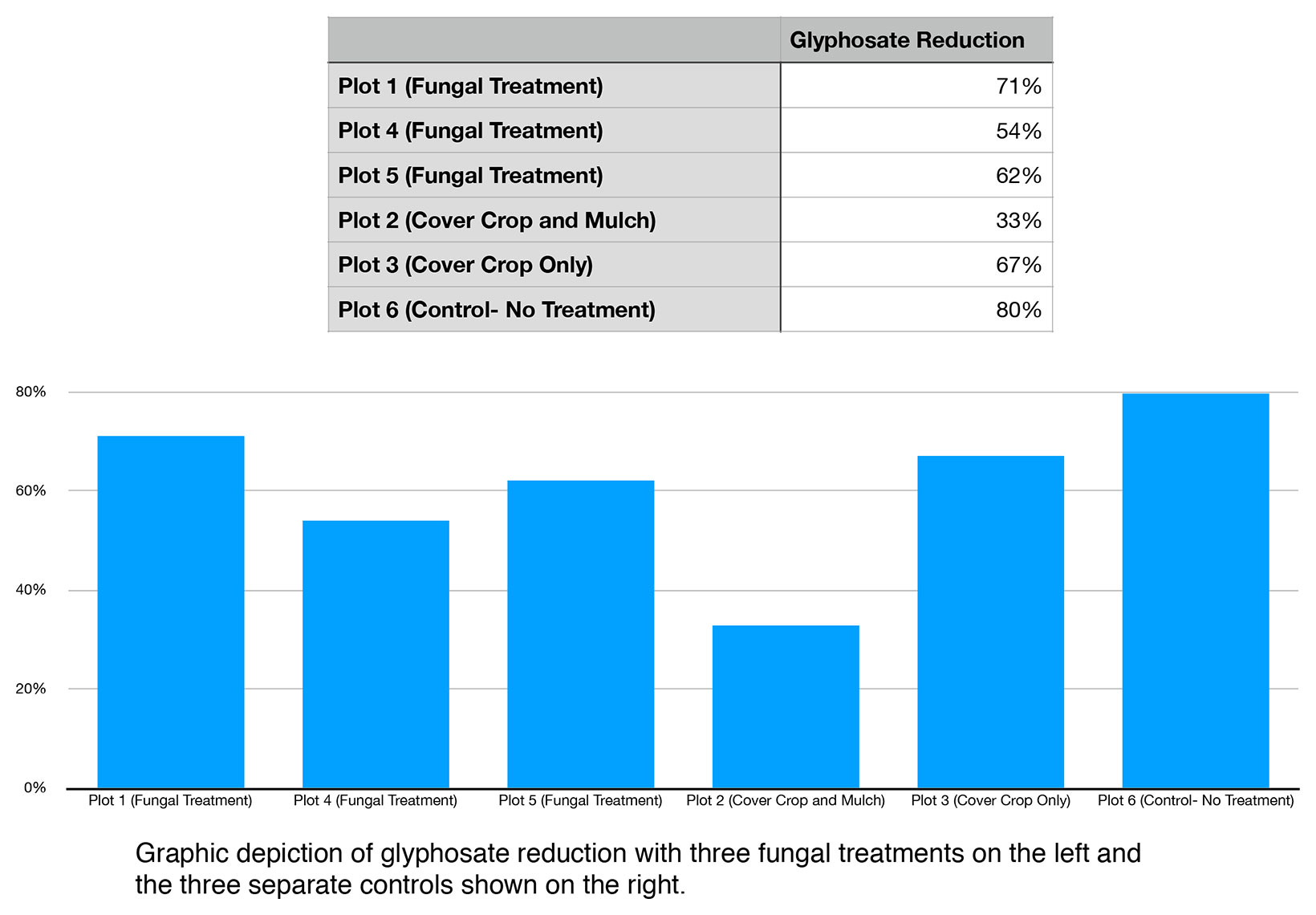
Figure 5. Percent glyphosate reduction.
When evaluated on the basis percentage reduction over time, the greatest reduction of AMPA occurred in Control Plot 6 (no treatment) and Fungal Treatment Plot 1 with an 51% reduction. The lowest rate of reduction was in Control Plot 2 (cover crop and rice straw/hulls mulch) with a 19% reduction. The average rate of reduction across all fungal treatments for AMPA (plot 1,4, and 5) was 36% (standard deviation of 13%), whereas the average for controls (plot 2, 3, and 6) was 39% (standard deviation of 17%).
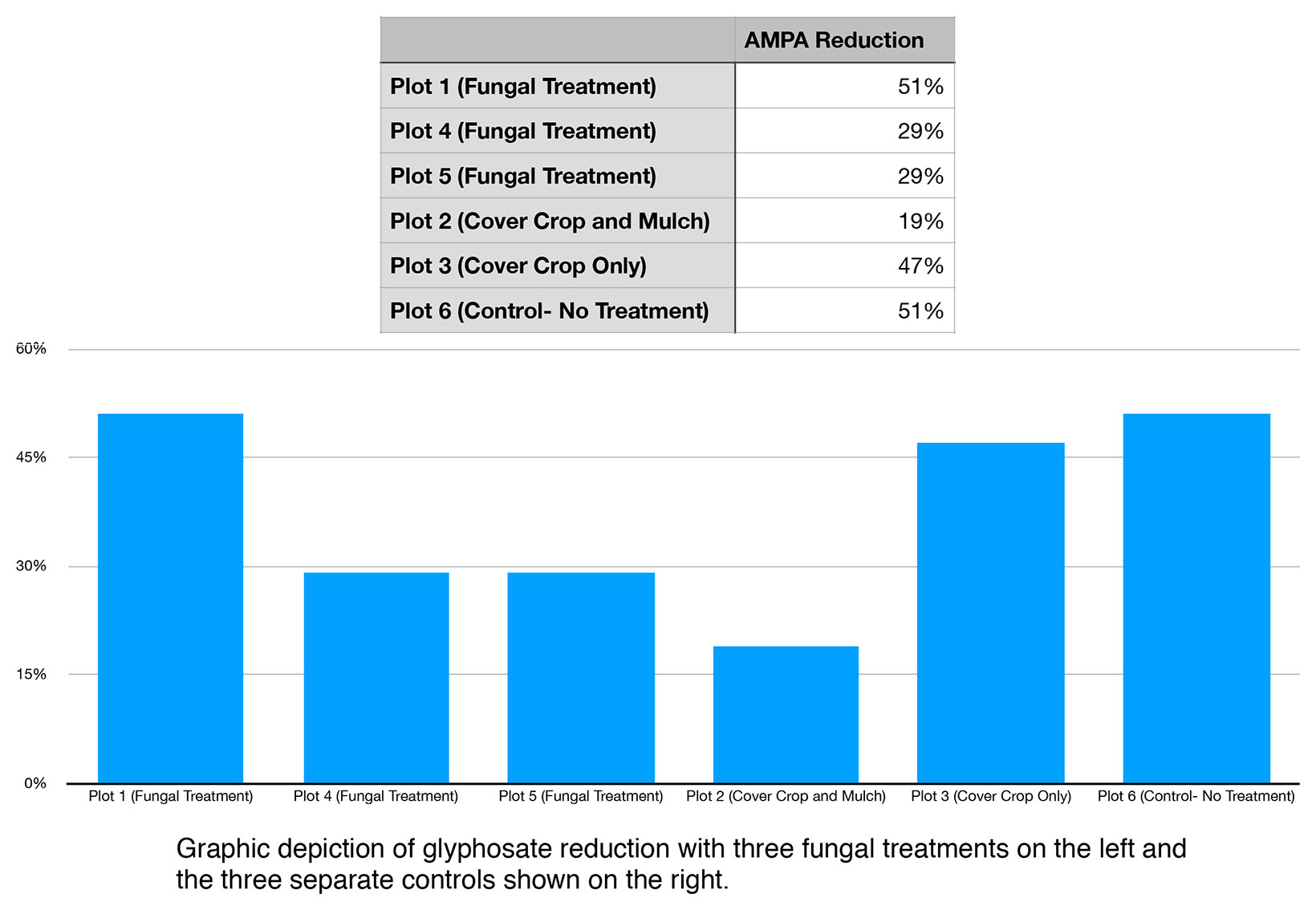
Figure 6. Percent AMPA Reduction.
Conclusions
On the most fundamental level, this study some indication about real-world lifespan of glyphosate and AMPA. While the glyphosate applications were not listed in the spray report, it is likely they occurred in the middle of the growing season 2019. For this study, soils were sampled in July of 2020. With samples taken a full year later, soil testing demonstrated the presence of glyphosate and AMPA after a 12 month period. With glyphosate still present a full year after application, this further questions the persistence of the pesticide. While many early studies suggest chemical half-life of glyphosate of 2-200 days, the initial samples taken in this study would indicate a much longer lifespan.
As for the effectiveness of fungals enzymes on pesticides, while there is strong literary support for fungal biodegradation of pesticides in-situ, that was not observed in this research. While glyphosate/AMPA reductions were observed across all plots, there was no clear and apparent correlation to fungal treatments being more effective than treatments. When evaluated on a percentage reduction basis, Control Plot 6, which received no tillage, no amendment, no cover crop and no irrigation had a greater than or equal to reduction for both glyphosate and AMPA.
In the circumstance Glyphosate, Control Plot 6 (No Treatment) demonstrated the greatest rate of reduction reduction (80%) verses any of the Fungal Treatment (Plots 1, 4, and 5 with 71%, 54%, and 62% reduction) respectively. Likewise Control Plot 6 (No Treatment) had a equal to (51%), or better than, reduction of AMPA, verses Fungal Treatments (51%, 29%, and 29% in Fungal Treatment Plots 1, 4, and 5 respectively).
The lowest rate of reduction for both glyphosate and AMPA was observed in Control Plot 2 in which 0.5 yards of rice straw/hulls had been tilled into the soil, cover crop had been seeded, and irrigation had been applied. In the circumstance of Control Plot 2 rates of reduction were 33% for glyphosate and 19% for AMPA. The purpose of this particular control was to account for the possibility that adding organic matter would inherently reduce the rate of pesticide contamination in soil samples, that is, the addition of material would yield lower pesticide rate based strictly due to a dilution of the native soil. The concept was that since 0.5 cu. yd. of organic matter was being added to the Fungal Treatments, one of the Control Treatments should receive the same volume of non-fungal organic matter to provide a fair comparison. To this same end all inputs used were certified organic to ensure no pesticide residues were imported on amendments, skewing results in the other direction.
Interestingly, the Control Plot 2 (cover crop and mulch) had the lowest rate of pesticide reduction while the totally untreated Control Plot 6 (no treatment) yielded the highest rate of reduction. Based on this, this author conjectures that cover cropping and mulch may protect glyphosate and AMPA from chemical oxidation while the area of the field left unirrigated and exposed to full sun may afford some sort of degradation in a high heat/oxygen environment.
It is also worth noting that Fungal Treatment plots 1, 4, and 5 are fundamentally identical to Control Plot 2 (cover crop and mulch) in treatment, except for the fact that a healthy fungal biology was introduced. So while Fungal Treatment Plots did not show reduction rates as high as the Untreated Control Plot, Fungal Treatments still performed significantly better than the uninoculated Plot 2.
That is to say, leaving a transitional field fallow and uncovered may lead to a more rapid amelioration of pesticide residues than cover cropping and mulching, but fungi could help speed glyphosate degradation in a system where we choose to employ the best management practices of cover cropping and organic matter additions. Another way of saying this is bare soil fallow may be more effective in glyphosate reduction than a cover crop, amended with organic matter system, but a cover crop amended with fungi is better than one without the addition of biology (bare soil > cover crop/mulch < cover crop/mulch/fungi < bare soil).
Future work could replicate this study with the addition of several Untreated plots to determine if the rates of reduction in the Untreated Plot was a datum anomaly or wether bare soil fallow can degrade pesticide residues faster than cover cropping with organic matter additions. In the sustainable agriculture community it is widely understood that cover cropping is a basic tool in increasing organic matter, improving tilth and bolstering biology. That said, glyphosate residues in transitional systems inhibits metabolic pathways in plants, reduces the nutritional value of crops, and have potential health effects for the end consumer. The question could be posed; in a transitional system is it best to leave a field in bare-soil fallow for its first season before employing best management cover cropping systems.
Additional work needs to be done in scalable fungal compost applications for larger organic systems. Fungal based composts and compost teas are currently in-vogue within the organic farming community (i.e. Johnson-Sou/B.E.A.M compost). Low tech mushroom cultivation techniques offer a much more rapid and scalable option to generating fungi on a much shorter time frame. Development of a standard operating procedure for fungal culture that is simple and requires little infrastructure would help fungal-based compost enthusiasts improve their programs.